Down House, Charles Darwin’s family house in which Darwin lived and worked, wrote On the Origin of Species and conducted many elegant experiments to test his ideas. Down House is now preserved for public viewing as a legacy of Darwin’s enduring influence on science and human affairs. Photo: Geetha Gadagkar
- ‘Survival of the fittest’ willy-nilly implies a perfect body that is incompatible with a body with “a thousand flaws and frailties that make it vulnerable to disease”.
- Indeed, ‘survival of the fittest’ – a phrase originally used by Herbert Spencer and later somewhat uncritically adopted by Darwin – is rather misleading.
- We now understand that natural selection maximises the ability of organisms to leave behind copies of their genes in the bodies of future generations.
- Our bodies are both “extraordinarily precise and unbelievably slipshod”, yet this is what we should expect as products of evolution by natural selection.
- An emerging discipline, evolutionary medicine, provides several explanations to this and shows how to use their wisdom in preventing and treating disease.
In the late 1990s, I came across a book by Randolph M. Nesse and George C. Williams published in the US with the provocative title Why We Get Sick. The alternate title of the UK edition, Evolution and Healing: The New Science of Darwinian Medicine, promised a scientific, evolutionary answer to the question raised by the US title.
Ever a sucker for clever and witty remarks, I was seized by a blurb: “Buy two copies and give one to your doctor,” by Richard Dawkins. I did not know who Randolph Nesse was, but I knew that George Williams was a famous evolutionary biologist best known (at least to me) for his excellent book, Adaptation and Natural Selection (1974), a strong critique of sloppy thinking by laypeople and specialists alike about how natural selection works. I bought one copy (to start with) and began to read on the bus home. The first paragraph said:
“Why, in a body of such exquisite design, are there a thousand flaws and frailties that make us vulnerable to disease? If evolution by natural selection can shape sophisticated mechanisms such as the eye, heart and brain, why hasn’t it shaped ways to prevent nearsightedness, heart attacks, and Alzheimer’s disease? If our immune system can recognize and attack a million foreign proteins, why do we still get pneumonia? If a coil of DNA can reliably encode plans for an adult organism with ten trillion specialized cells, each in its proper place, why can’t we grow a replacement for a damaged finger? If we can live a hundred years, why not two hundred?”
As I read on, the irony gradually dawned on me. The ‘failures’ of natural selection described in the first paragraph were the strongest arguments in favour of thinking of our bodies as having been shaped by evolution by natural selection! No divine creator or intelligent designer could have bungled so badly. In the words of Nesse and Williams:
“The design of our bodies is simultaneously, extraordinarily precise and unbelievably slipshod. It is as if the best engineers in the universe took every seventh day off and turned the work over to bumbling amateurs.”
On the other hand, if we are the product of evolution by natural selection, these are precisely the kinds of flaws and vulnerabilities we should expect. But why?
An emerging new discipline, evolutionary medicine, provides several answers to this question and shows how to use their wisdom in preventing and treating disease.
The birth of a new discipline
Most scientists consider themselves too busy to pay attention to the history of science, let alone to its sociology and philosophy. Indeed, an interest in these disciplines, especially in young scientists, is often dismissed as a sign of a lack of motivation or inability to get on with the serious job at hand. And yet, we learn so much from history, both how to avoid past mistakes and how to do good science, even in the face of adversity. So it is worth wondering how the discipline of evolutionary medicine was born.
The story goes that Randolph Nesse, a physician in the Department of Psychiatry at the University of Michigan Medical School, was “frustrated with psychiatry’s lack of theoretical foundation” on the one hand and “fascinated with the extraordinary progress that evolutionary ideas had brought about to the field of animal behavior” on the other. This led him to hang out with colleagues interested in evolution and human behaviour, who suggested he get in touch with a biologist named George C. Williams, who had proposed an evolutionary explanation for aging. Nesse complied, arguing that “if aging had an evolutionary explanation, why not anxiety disorders or schizophrenia?”
George C. Williams, who wrote the 1957 paper Pleiotropy, Natural Selection, and the Evolution of Senescence, was a marine ecologist in the Department of Ecology & Evolution at the State University of New York. Williams traces the origin of his interest in the application of evolutionary thinking in medicine to a paper by the ornithologist Paul Ewald at the University of Washington. Published in the Journal of Theoretical Biology in 1980, Ewald’s paper had a rather explicit title: Evolutionary Biology and the Treatment of Signs and Symptoms of Infectious Disease. In this paper, Ewald argued that:
“When viewed from an evolutionary perspective, manifestations of infectious diseases can be classified as:
(1) adaptations of the host to counteract harmful aspects of the disease,
(2) adaptations of the pathogen to manipulate the host, or
(3) “side effects” of the disease that do not serve adaptive functions for either the host or the pathogen.”
But he ended cautiously by noting, “This approach should lead to improved medical treatment because preferred treatment depends on assessment of the validity of the three explanations.”
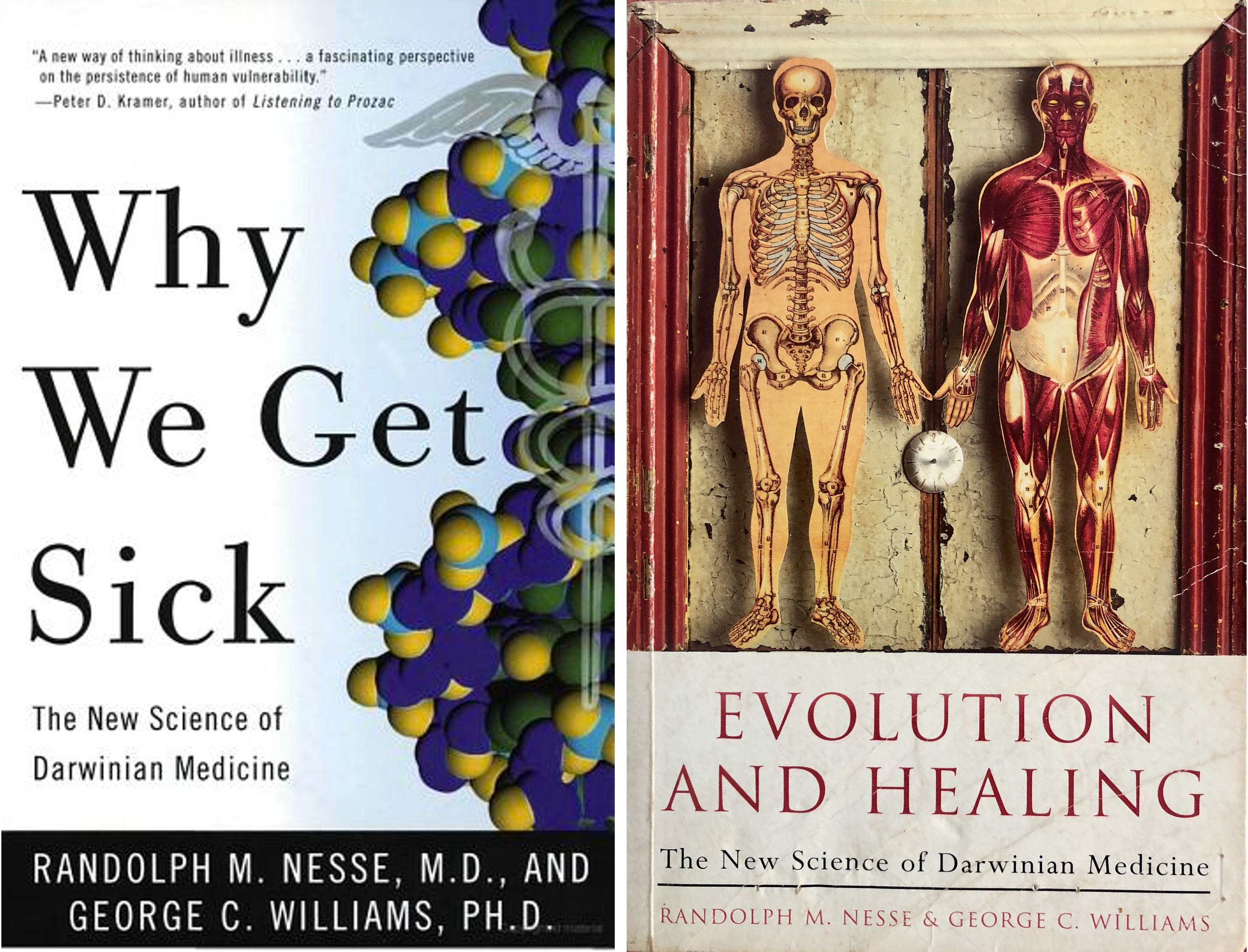
We see in retrospect that in an effort seemingly quite outside his main area of expertise, Paul Ewald made a lasting contribution to the birth of a new discipline – a contribution that was arguably more important than his outstanding work in ornithology. Indeed, evolutionary medicine can be said to have had three parents: an ornithologist (Ewald), a psychiatrist (Nesse) and a marine biologist (Williams). Such unlikely parentage is a recurring pattern in the history of scientific ideas, but one that conservative and unimaginative assessment and evaluation procedures in academia can easily nip in the bud.
With his interest in the evolution of aging, Williams was already primed to appreciate Ewald’s insights. And for the reasons described above, Nesse was already primed to collaborate with Williams in the pursuit of evolutionary interpretations of health, disease and medicine.
Evolutionary insights into health and disease
Ewald’s classification of the manifestations of infectious diseases makes it obvious that we should not indiscriminately attempt to mitigate all of them. For example, we may do more harm than good if we try to eliminate those that may be the body’s adaptations to combat the disease.
Nesse and Williams considerably broadened the scope of evolutionary thinking in the realms of health and disease. To test the waters, as it were, they first published a review article in the Quarterly Review of Biology in 1991, which announced (via its title) The Dawn of Darwinian Medicine. Making it explicit that their goal was not merely to formulate evolutionary insights into disease but to get doctors to do so, they began their paper with the following claim:
“Medical education stresses physics, chemistry, and those branches of biology that deal with proximate mechanisms. Application of this knowledge to biology and medicine has resulted in impressive advances in the prevention and treatment of human disease. Evolutionary biology, however, has not been emphasized in medical curricula. This is unfortunate, because new applications of evolutionary principles to medical problems show that advances would be even more rapid if medical professionals were as attuned to Darwin as they have been to Pasteur.”
This was a strategic move because they realised that evolutionary medicine was not yet a mature body of knowledge waiting to be transferred to doctors, but a new way of thinking that could yield significant benefits if physicians themselves adopted it. So their task was to sell the idea of evolutionary thinking to doctors, whetting their appetite with some preliminary insights.
At the outset, Williams and Nesse make a necessary correction to the popular conception of natural selection indicated by the phrase ‘survival of the fittest’ to pave the way to understanding how natural selection can sometimes make us less fit.
‘Survival of the fittest’ willy-nilly implies a perfect body that is incompatible with a body with “a thousand flaws and frailties that make it vulnerable to disease”. Indeed, ‘survival of the fittest’ – a phrase originally used by Herbert Spencer and later somewhat uncritically adopted by Darwin – is rather misleading.
We now understand that natural selection maximises the ability of organisms to leave behind copies of their genes in the bodies of future generations. This is sometimes called the gene’s eye view of evolution and was first suggested by G.C. Williams and later more explicitly by Richard Dawkins as the ‘selfish gene’ concept. It makes it possible to understand that maximisation of genetic representation may sometimes be better achieved without necessarily making the individuals long-lived, disease-free or resistant to bodily damage.
Moreover, of two competing individuals, the one that leaves behind more copies of its genes is favoured by natural selection, even though neither may be very fit in absolute terms.
It is important to recognise the multiple meanings of the terms ‘fit’ and ‘fitness’. In common parlance, we mean strong and free from disease, free from “a thousand flaws and fragilities” when we say ‘fit’. Let us call this the doctor’s definition of fitness. Until recently, evolutionary biologists measured fitness as the total number of offspring produced in one’s lifetime, the so-called lifetime reproductive success (LRS). Let us call this classical Darwinian fitness.
More recently, evolutionary biologists have realised that a form of natural selection called kin selection may favour traits that make individuals produce fewer direct offspring but help in the care and survival of close genetic relatives. This is because genetic relatives also carry copies of our genes. So the sum of the number of offspring plus a measure of one’s contribution to the survival of relatives has come to be known as inclusive fitness.
Note that the fitness in the title of this article is the common sense or doctor’s definition of fitness. And the point of the article is that classical Darwinian fitness or inclusive fitness may sometimes be maximised despite low fitness in the doctor’s sense of fitness. Indeed, as we shall see, low fitness in the doctor’s definition is sometimes an inevitable consequence of the process of natural selection working towards maximising classical Darwinian or inclusive fitness!
Thus, the gene’s eye view of evolution and understanding the distinction between the doctor’s definition of fitness and the evolutionary biologist’s definition of fitness prepares the ground to apply evolutionary reasoning to the problems of health and disease. In their book, Why We Get Sick, Nesse and Williams discuss five reasons we are frail, vulnerable and prone to disease, despite or, indeed because of, the action of natural selection.
The curse of history
Many of the apparent design flaws in our bodies owe their existence to the fact that natural selection is a process of differential survival of alternative phenotypes that compete with each other at a given place or time. The better of the two wins no matter how bad it is in absolute terms. Thus, when organisms move to a different environment, natural selection favours those in which small mutations make them even slightly better adapted than the others.
Natural selection simply makes the best of a bad job. It has no way of going back to the drawing board and starting from scratch, as an engineer might do. Moreover, every intermediate stage must be at least as good, if not better, than the previous one, putting enormous constraints on the path from good to better. This is why we figuratively say that natural selection has no foresight; it cannot today favour the inferior of the variants because that will be much better in the future. Natural selection is a blind, mechanical process acting here and now.
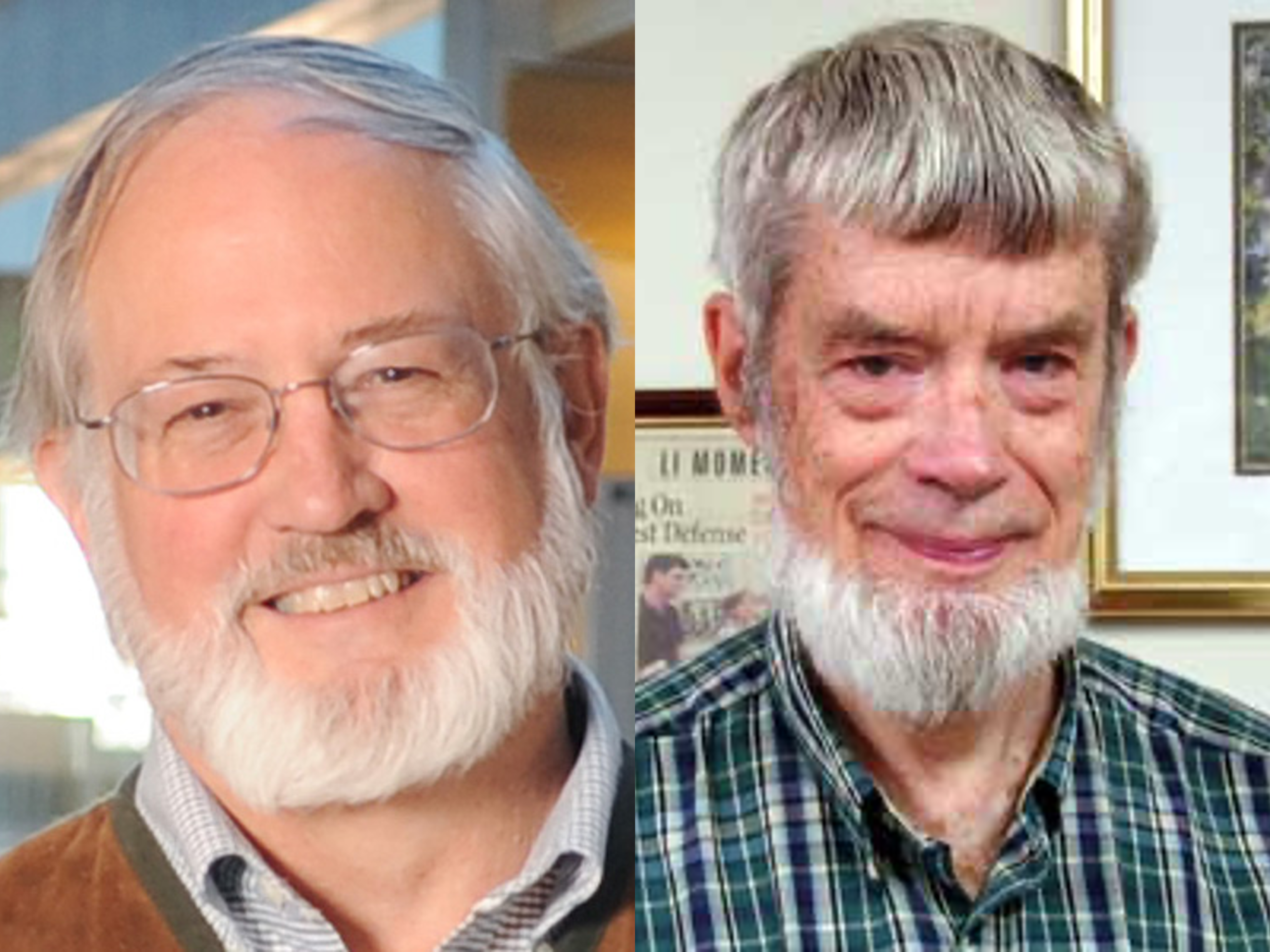
Perhaps the best-known design flaw because of the curse of history, technically called phylogenetic inertia or phylogenetic constraint, is the one that makes us choke on our food or drink. Even Darwin noted:
“… the strange fact that every particle of food and drink which we swallow has to pass over the orifice of the trachea, with some risk of falling into the lungs, notwithstanding the beautiful contrivance by which the glottis is closed.”
The reason for this is that we have ‘descended with modification’, to use Darwin’s favourite phrase – from minute worms that fed and breathed by filtering small particles of food from the water that entered their bodies, as they also absorbed the oxygen in the water. When the descendants of these worms began to breathe air, a passage was created from the existing digestive system to carry air to the lungs. Along with all vertebrates, we are stuck with the design flaw of crisscrossing air and food passages, making us vulnerable to choking; about one in a hundred thousand persons are estimated to die of this cause every year.
Given the phylogenetic constraint, that we have descended from filter-feeding worms via a series of slight modifications, each one making us slightly better than our ancestors, there is no way that natural selection could have solved this problem by creating two separate passages for food and air – as it has done in the case of insects and molluscs.
Host-parasite arms races
An evolutionary perspective makes clear why natural selection cannot eliminate all parasites and make us disease-free. The simple fact is that the same process of natural selection is also working constantly to make our disease-causing parasites as successful as possible. Thus, we are in a constant arms race with pathogens. And in many ways, pathogens have the upper hand over us in utilising the power of natural selection for self-improvement.
Since natural selection works by the differential reproduction of alternate phenotypes, the rate of evolution is a function of the number of generations, not absolute time. Since parasites typically reproduce much faster than their hosts, they can counter the strategies of their hosts much faster than the hosts can retaliate. Most disease-causing parasites go through hundreds if not thousands, or even millions, of generations in our bodies, and we can only experience any evolutionary change to combat them in subsequent generations.
We are therefore sitting ducks for the parasites to evolve strategies to counter our previously evolved defences.
It is believed that sexual reproduction itself evolved to combat parasites: by combining genes from two parents and shuffling our genes through recombination, we present the parasites in the environment with a new gene combination they are not used to. They will have to start all over again to counter our defences, and we thus buy some time to mount immune and other defences against them. In recent times, we have realised that parasites not only rapidly evolve defences to our naturally evolved anti-parasite strategies but also to our technological strategies, such as the antibiotics we use against them.
An evolutionary perspective on host-parasite interactions offers us a new understanding of disease as well as suggests completely novel and counterintuitive policy prescriptions about how to deal with disease. Recall Ewald’s classification of the symptoms of infectious diseases and the resulting idea that we should be cautious about mitigating the symptoms of a disease without understanding that some of them are our body’s defence mechanisms. We should complement our body’s own efforts and not work against them.
This requires not merely evolutionary knowledge, but also evolutionary wisdom. Doctors must become more modest and realise that medical intervention is often only a small augmentation of the body’s defences evolved over millions of years.
Why fever?
Why, for example, do we get a fever when we get an infection? There is growing evidence that in all homeotherms (warm-blooded animals), fever is the body’s adaptive response to infection and not a malfunction of the mechanism of thermoregulation. So treating a fever may interfere with the body’s attempts to fight the disease and may do more harm than good.
There is a surprising paucity of good research on the costs and benefits of fever. Realising this, Nesse and Williams show abundant caution in calling for more research rather than making an outright recommendation that doctors refrain from treating fevers. They are clear that they
“… would not dream of recommending that people never take drugs to reduce fever. Even if many studies were to establish decisively that fever is usually important for combating infection, that would not justify an unbending policy of encouraging fever or even of routinely letting it rise to its natural level.
An evolutionary perspective draws attention to the costs as well as the benefits of an adaptation like a fever. If there were no compensating disadvantage in having the human body operate at 40º C. (103º F.), it ought to stay at that temperature all the time, so as to prevent infections from ever getting started. But even this moderate fever has costs; it depletes nutrient reserves 20% faster and causes temporary male sterility. Still higher fevers can cause delirium and perhaps seizures and lasting tissue damage.
It should also be realized that no regulation mechanism can perfectly anticipate all situations. We would expect temperature to rise, on average, to a level close to an optimum to fight infection, but because regulatory precision is limited, fever will sometimes rise too much and at other times not enough.”
Similar arguments can be made about anaemia, which may result from the body’s adaptive defence against infection – in the attempt to restrict the availability of much-needed iron to the bacteria.
Clearly, more research is needed, but medical researchers are unlikely to be motivated to investigate the adaptive significance of fever or anaemia and the limits of such adaptation unless evolutionary biology becomes part of their basic education. When they study evolutionary biology and understand it as a way of integrating all of biology, they are more likely to become aware of the body’s evolved defence mechanisms and begin to see themselves as partners in the body’s efforts to combat disease.
Thus, natural selection seems to make us ill (or seemingly less fit) because it also works on behalf of disease-causing pathogens and because a certain amount of temporary illness may be necessary to combat the disease. Therefore, the recognition that pathogens are also being favoured by natural selection to become better at what they do and that some of the symptoms of disease may be the result of the body’s defence mechanism have the potential to profoundly alter both our conception of disease as well as its treatment.
A mismatch
A third reason natural selection may render us seemingly unfit is a mismatch between the environment in which we evolved and the environment that we experience today.
It is often said that our modern culture does not match our stone-age biology. Unfortunately, such a presumed mismatch is one of the most misused concepts in evolutionary biology, being applied indiscriminately to explain away all our problems. What is worse is that some people argue that we must therefore abandon our modern lifestyle entirely and go back to living as we supposedly did in the golden stone age. It is this kind of careless and casual application of evolutionary biology that gives it a bad name and leads to much harm.
This said, when rigorously applied with sufficient evidence, the concept of mismatch is indeed an accurate and helpful idea in understanding and dealing with some of our current ailments. In a fascinating and comprehensive book-length treatment entitled Mismatch (2008), Peter Gluckman and Mark Hanson showed that a mismatch between our evolved biology and our current environment can occur for many reasons.
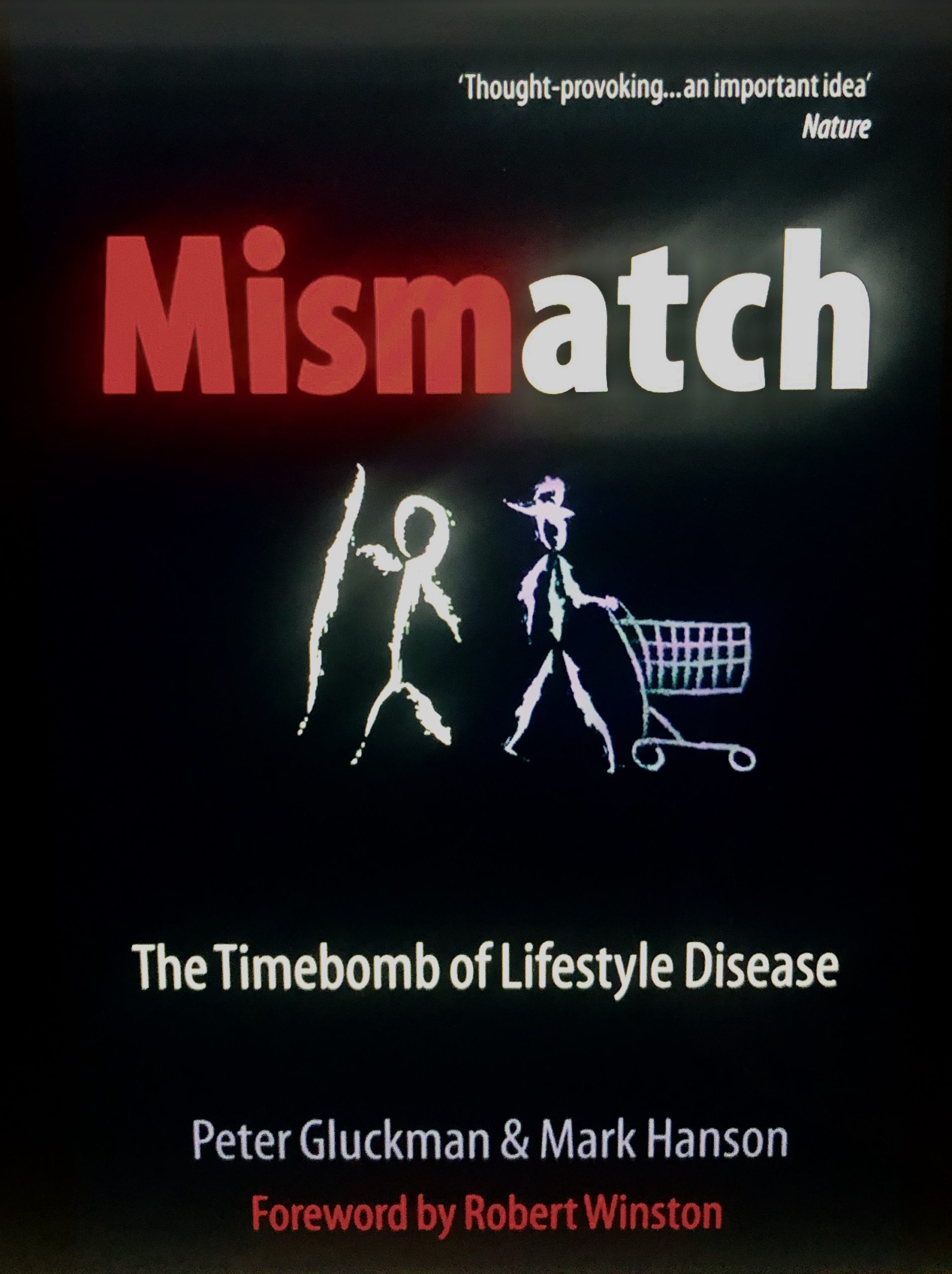
For example, in modern western societies with access to better nutrition and healthcare, the timing of psychosocial and sexual maturity, which used to occur more or less simultaneously in the past, have now become delinked. Children nowadays reach sexual maturity relatively early and before they have reached the psychosocial maturity required to deal with the demands of sexual maturity, leading to the many problems adolescents face today.
Another example is the mismatch between our biology on the one hand and our modern lifestyle of diets, energy consumption and expenditure on the other. With the advent of agriculture and modern technology, our pattern of food intake has changed dramatically. Fats, salt and sugar, which were very scarce, have now become abundantly and predictably available. This much is because of advances in agriculture and technology. Marketing strategies and business interests in manipulating people into consuming more than they need substantially exacerbate the problem. The net result is a suite of so-called lifestyle diseases such as obesity, type 2 diabetes, hypertension and cardiovascular disease.
But the links between diet and health are far from clear. Frequent U-turns in the recommendations for and against consuming foods such as red meat, eggs, fats and sugars are all too familiar. They often result in the loss of trust in science not only among the general public but also among scientists in other disciplines. The truth of the matter is that these links are mindbogglingly complex, and much more research is needed, especially keeping an evolutionary perspective in mind.
In fact, since the recommendations for and against the consumption of different foods are loaded with strong commercial interest, the very nature of scientific research and its evaluation are at stake. A recent editorial in Nature, entitled ‘Studies linking diet with health must get a whole lot better’, highlighted that “the evidence base surrounding much nutritional and wider health advice is … disputed” and has concluded that “underpowered clinical studies, lacking necessary controls to make sense of the data, are not helping”. The editorial also warned:
“If funders do not target their efforts at producing quality data, the public will remain confused, weary, distrustful and deprived of the information they need to make informed health and lifestyle choices…”
Yet another mismatch occurs because people’s post-reproductive lifespans are getting longer than before, leading to a host of old age diseases, including dementia, Parkinson’s disease, Alzheimer’s disease and complications associated with menopause. We are not adapted to these because they are relatively more recent and because old age itself is now more common than it was before.
What is worse is that since these diseases occur only or mainly in old age, natural selection can’t even select against them, because they do not affect our reproductive success, making an evolutionary perspective even more crucial.
As we have seen above, many cases of mismatch occur because natural selection is yet to catch up with our modern culture. It is ironic that natural selection, which has given rise to our large brains and the capacity for language and thus facilitated the development of culture, has let us escape from the power of natural selection itself. An obvious question is whether it can catch up in the long run. The answer seems to be ‘yes’ in principle, but in practice, ‘probably not’, or ‘only very rarely’.
The goodness of milk?
The best-studied example of a culture-induced mismatch of our biology and environment where the gap may eventually be closed is that of lactose tolerance/intolerance. Since all mammals suckle their babies with milk from the mother, babies have the enzyme lactase that breaks down the milk sugar lactose.
After the babies are weaned and switched to other diets, they stop making lactase, which is no longer needed. Humans do the same. Adults are thus technically labelled ‘lactase non-persistent’ and therefore ‘lactose intolerant’. But with the advent of animal husbandry in some parts of the world, it became very profitable to include animal milk as a part of our adult diet.
There is good evidence that natural selection favoured rare mutations that allowed individuals to continue synthesising the enzyme lactase throughout adulthood and become ‘lactase-persistent’ and, thus, ‘lactose tolerant’. There is also evidence that several different mutations leading to the same outcome have been selected in different parts of the world. The net result is that lactase persistence and the associated lactose tolerance are now common in many parts of Europe and some parts of Africa and the Middle East.
However, its prevalence is highly variable, ranging from 95-97% in Scandinavia, where milk consumption is common, to 0-10% in East Asia, where milk consumption is rare.
On the whole, lactose intolerance remains by far the most common condition in the world, and indeed it is the ancestral condition in humans. But due to the lack of an evolutionary perspective and an all too familiar western bias, we often consider lactose intolerance to be a disease.
An evolutionary perspective lets us see a very different picture. From the ancestral condition of lactose intolerance, when milk consumption was absent, to the present day when milk consumption is common, at least in some parts of the world, lactose tolerance has evolved, reversing the mismatch between biology and culture. It is reasonable to expect that lactose tolerance will similarly evolve if milk consumption spreads to other parts of the world.
But of course, natural selection is a slow process, and such mismatches are expected to persist for hundreds, if not thousands, of years.
Blame it on the genes
Like many attributes of our bodies, many of our diseases can also be blamed on our genes. But this seems counterintuitive because eliminating such genes is precisely the job of natural selection. The problem is that natural selection is sometimes powerless to do so; it can’t, for example, eliminate bad genes that may have some good effects.
The best-known example is the gene that causes sickle-cell anaemia. This gene, which is relatively common in some parts of Africa, produces abnormal haemoglobin that causes the red blood cells to be deformed and assume the shape of a sickle. Such sickle cells cannot circulate properly in the blood, leading to bleeding, anaemia and many associated problems.
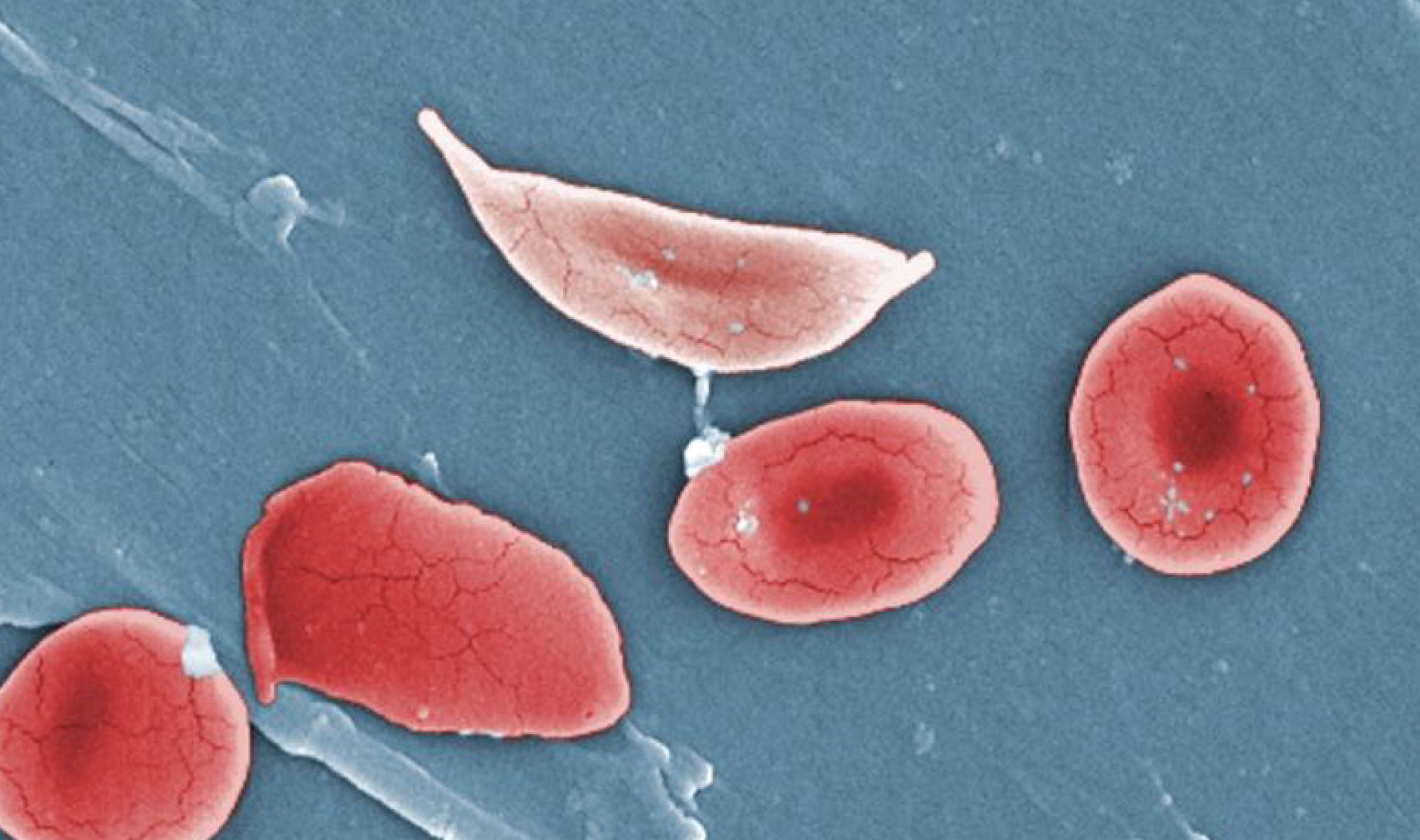
If all the red blood cells in the body are sickled, as it happens when individuals carry two copies of the defective gene (inherited both from the mother and the father), then such individuals (referred to as homozygous for that gene) are severely diseased. They usually die before they become reproductively mature. If this was the whole story, natural selection could have eliminated the sickle cell gene from the populations. But there is a quirk.
Individuals who inherit the defective gene from one parent and a normal gene from the other parent are heterozygous, i.e. they have one normal and one defective gene. In these individuals, the normal gene produces normal haemoglobin and normal red blood cells, and they can make do with these normal cells. The abnormal red blood cells produced by the defective gene are removed from the bloodstream. Thus, the sickle cell gene needs both copies in the body to be defective to manifest the disease, and such genes are called recessive.
Natural selection has a more formidable challenge to eliminate recessive lethal genes because they hide from natural selection in the heterozygotes, who manage to survive despite carrying one defective gene, and pass on the defective gene to the next generation. If the gene is very rare in the population, chances are that most carriers are heterozygous, and natural selection becomes even less effective. Hence, recessive lethal genes persist in the population at a low frequency for a very long time.
But imagine that due to some quirk, the heterozygotes actually fare better than those who are homozygous for the normal gene. Can this happen? Yes, it can and does under some circumstances, for e.g. in the case of the sickle-cell gene.
Because heterozygous individuals manage by eliminating the defective red blood cells, they make life difficult for the malarial parasite Plasmodium falciparum, as the red blood cells carrying them are often eliminated. Thus, heterozygotes for the sickle cell gene have considerable protection from the risk of death from malaria.
In effect, the sickle-cell gene in the body of the heterozygote is not only protected from elimination by natural selection but actually favoured. As expected, the sickle-cell gene is more common in malaria-prone areas, notwithstanding the fact that individuals homozygous for the gene are born and suffer from the disease – a situation that natural selection is powerless to correct as long as malaria is prevalent.
Our bodies are a bundle of trade-offs
There is yet another reason why natural selection may be constrained in its ability to perfect the adaptations of organisms to their environment. In perfecting some features of our body for one function, there is often a trade-off with another. You can’t have your cake and eat it too. As Williams and Nesse say:
“Walking upright has a price in back problems. The capacity for tissue repair has a price of cancer. The immune response has a price of immune disorders. The price of anxiety is panic disorder. In each case, natural selection has done the best it can, weighing benefits against costs. Wherever the balance point, however, there will be disease. The adaptationist does not view the body as a perfect creation, but as a bundle of compromises. By understanding them, we will better understand disease.”
In future articles, we will explore more trade-offs, including those between youth and old age and between males and females.
***
In summary, our bodies are frail and faulty and prone to disease because natural selection may have taken certain historical trajectories, because natural selection also simultaneously works on behalf of disease-causing pathogens, because there is a mismatch between our biology and our culture, because bad genes can have some good effects or because there are often trade-offs that limit its ability to perfect several functions simultaneously.
For an evolutionary biologist, this is exciting basic science dazzling us with its sheer beauty and logic. But there can be more. The promise of evolutionary medicine is that viewing our bodies as having evolved by natural selection and understanding the reasons behind health and disease from an evolutionary perspective will strengthen medical science.
As basic scientists, we must be careful, however, in making claims about the practical application of our knowledge. I was therefore impressed with the abundant caution and modesty with which Nesse and Williams, a psychiatrist and a marine biologist, venture to advise doctors. They admit upfront in the preface to their book that:
“Darwinian medicine is still in its infancy… We have every expectation that the pursuit of evolutionary questions will demonstrably improve human health. That will require effort, money, and time. In the meanwhile, we hope this book will stimulate people to think about their illnesses in a different way, to ask questions of their doctors, perhaps even argue with them, but certainly not to ignore their instructions.”
In the next article, we will take stock of the present state of evolutionary medicine, some 30-35 years after the publication of Nesse and Williams’s bold and pioneering work.
Raghavendra Gadagkar is a Department of Science and Technology (DST) Year of Science Chair Professor at the Centre for Ecological Sciences at the Indian Institute of Science, Bengaluru.