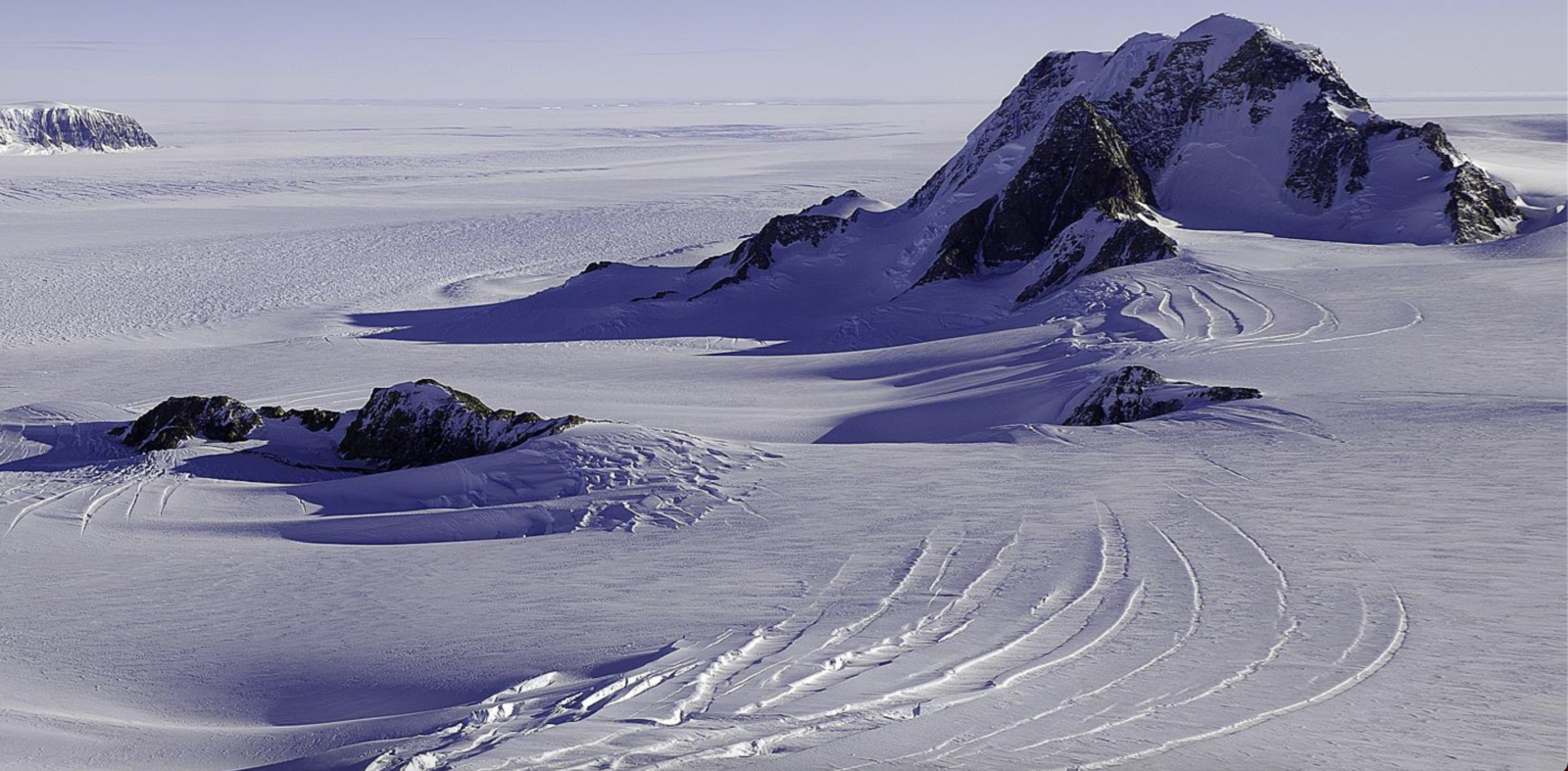
A view of Marie Byrd Land in West Antarctica, 2011. Photo: NASA/Michael Studinger
- Higher seas will cause shorelines to recede and coastal flooding to worsen impacts on communities, infrastructure, natural resources and biodiversity on the world’s coastlines.
- It is already unavoidable that many communities will be displaced and, in some cases, forced to migrate as climate refugees.
- Quantifying the pace of global mean sea level rise as well as the magnitude of the long-term rise is thus essential for effective adaptation planning and the evaluation of mitigation pathways and policies.
- Pinning down these quantities requires a focused effort from the scientific community to understand the key rate-determining processes that affect melting of the Antarctic ice sheet.
Among the most visible effects of anthropogenic global warming are rising seas around the world: since 1880, the global mean sea level (GMSL) has increased by 20 centimeters. There’s no easy way to halt or reverse this change. Earth’s ocean and ice sheets respond slowly to changes in the heat they receive from the atmosphere, and they hold onto heat for decades to centuries. As a result, sea level globally will continue to rise well beyond the 21st century, even if warming of the planet is stabilised below the target set by the Paris climate agreement in 2015 of 2º C above the preindustrial average.
The recent Intergovernmental Panel on Climate Change (IPCC) Sixth Assessment Report (AR6) and the 2019 revision of the World Population Prospects both state that it is very likely that climate change-induced sea level rise will affect much of the world’s coasts in the coming decades. An estimated 800 million people are likely to experience impacts of high-tide flooding by the end of the 21st century, even if the Paris climate agreement target is met.
In many coastal settings, even a small increase in baseline sea level can substantially increase the frequency and magnitude of flooding during high tides, storm surges, and extreme weather. The UN estimates that the potential costs of damage to harbours and ports alone from this flooding could be as high as $111.6 billion by 2050 and $367.2 billion by the end of the century.
What’s more, if policies aimed at curtailing greenhouse gas emissions and atmospheric warming this century fail, sea level rise will accelerate, dramatically reshaping our shorelines for centuries to come. Higher seas will cause shorelines to recede and coastal flooding to worsen impacts on communities, infrastructure, natural resources, and biodiversity on all the world’s coastlines. It is already unavoidable that many communities will be displaced and, in some cases, forced to migrate as climate refugees.
Quantifying the pace of GMSL rise as well as the magnitude of the long-term rise (a few centuries onward), to which we are committed, is thus essential for effective adaptation planning and the evaluation of mitigation pathways and policies. Pinning down these quantities requires a focused effort from the scientific community to identify and understand the key rate-determining processes that affect melting of the Antarctic Ice Sheet (AIS) – the largest and most uncertain potential contributor to future sea level rise.
Many of these processes generate dynamical instabilities and involve nonlinear and potentially irreversible behaviors. Thus, establishing their relative roles in future ice sheet dynamics will not only improve sea level projections but may identify a threshold level of atmospheric carbon dioxide that once crossed, may cause unstoppable, multigenerational mass loss from the AIS and commitment to global sea level rise.
In February 2021, Instabilities and Thresholds in Antarctica (INSTANT), an international, interdisciplinary, and interorganisational program of the Scientific Committee on Antarctic Research (SCAR), was launched with the specific goal of reducing these uncertainties and filling gaps in our knowledge of the AIS.
Disappearing ice shelves
About a third of the AIS is marine based, resting on bedrock below sea level, and most of the ice sheet margin terminates in the ocean, making it susceptible to dynamical instabilities that can cause rapid ice loss (Pattyn and Morlighem, 2020). In many places around the ice sheet margin, the seaward-flowing ice forms floating ice shelves. Ice shelves in contact with bathymetric highs in the seafloor or confined within embayments provide buttressing that impedes the flow of upstream ice. Disintegration of ice shelves will therefore play a key role in the pace of future ice mass loss. Satellite observations show that most Antarctic ice shelves are currently thinning, primarily because of contact with warm subsurface ocean water (Adusumilli et al., 2020).
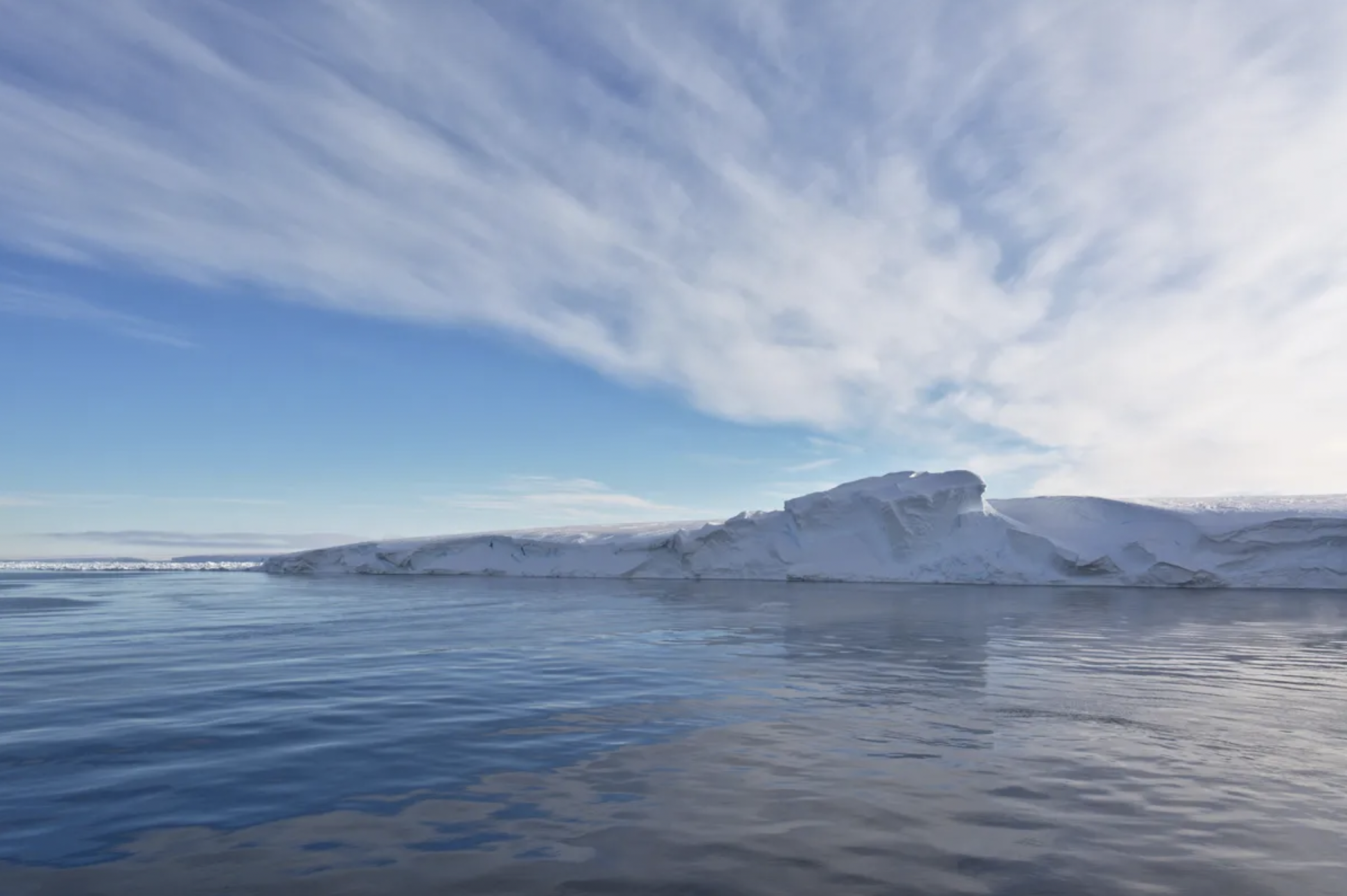
In the future, ice shelves could also become vulnerable to atmospheric warming and to the accumulation of surface meltwater, which can deepen crevasses and lead to sudden breakup through hydrofracturing. If the grounding line (the boundary between grounded and floating ice) is located on bedrock sloping down toward the ice sheet interior, the initial retreat caused by thinning ice shelves could result in a self-sustaining and potentially unstoppable process of retreat known as marine ice sheet instability (MISI).
Alternatively, disappearing ice shelves may lead to the formation of tall, unstable ice cliffs at the grounding line. Calving from these ice cliffs may then cause rapid ice sheet retreat by a process called marine ice cliff instability (MICI). It is possible that both types of instabilities could cause partial collapse of marine-based sectors of the AIS within a few centuries (DeConto et al., 2021).
Uncertain outcomes of complex processes
In addition to the effects of marine ice sheet and ice cliff instabilities, the rates of AIS mass loss and GMSL rise will be affected by complex interactions among ice, ocean, atmosphere, and solid Earth processes. These interactions involve both positive and negative feedbacks that amplify and reduce the rate of GMSL rise, respectively. For example, fresh water released as ice sheets thin and retreat reduces the formation of salty, dense Antarctic bottom water. Reductions in Antarctic bottom water weaken global thermohaline circulation, which is driven by differences in water temperature and salinity, leading to local and interhemispheric atmospheric cooling (Golledge et al., 2019). These reductions could ultimately reduce the pace of global warming and thus slow sea level rise (Golledge et al., 2019; DeConto et al., 2021).
However, fresh water released from ice sheets stratifies the surface ocean and subsequently enhances sea ice production, which disrupts the opening of sea ice–free areas called polynyas (e.g., Golledge et al., 2019), thus limiting gas and heat exchange between the atmosphere and the ocean. This sequence of feedbacks may focus warm seawater into cavities near grounding zones below ice shelves, increasing rates of ice loss (Silvano et al., 2018).
Critical steps to reduce uncertainties in the role of these processes and in projections of Antarctic ice loss involve elucidating the role of ocean dynamics by reconstructing deep- to near-past conditions, by observing present conditions, and by coupling numerical ocean circulation models to ice sheet models. These steps were among the most urgent research priorities to emerge from the IPCC Fifth Assessment Report (AR5), published in 2013, which predicted that the “likely” range of future carbon emission scenarios envisioned at the time would result in 28-98 centimeters of GMSL rise by 2100.
However, the AR5 estimates were limited by a lack of scientific understanding of key processes affecting dynamic loss of the AIS. Since then, the incorporation of instabilities such as MICI and MISI into numerical ice sheet models has resulted in a low-probability, high-impact future sea level rise scenario included in the recently released IPCC AR6, in which GMSL rise of up to 2 meters by 2100 “could not be ruled out” (see figure 1).
In general, the latest generation of numerical ice sheet models shows that the acceleration in mass loss observed by satellites over the past 10 years will continue (IMBIE team, 2018), although different models show a wide range of projections for Antarctica’s future contribution to GMSL rise because they treat the physics of potentially important processes in considerably different ways (e.g., Edwards et al., 2021) (see figure 1).
If global carbon emissions follow the high-emission Shared Socioeconomic Pathway (SSP) 5-8.5, meaning atmospheric carbon dioxide levels rise above 1,000 parts per million by 2100 (figure 1), melting Antarctic ice would contribute 14–32 centimeters (13th-87th percentiles) to an overall GMSL rise of 62-101 centimetres (relative to the 1995-2014 baseline) over the same period, according to a statistical assessment of numerical model projections (e.g., Edwards et al., 2021).
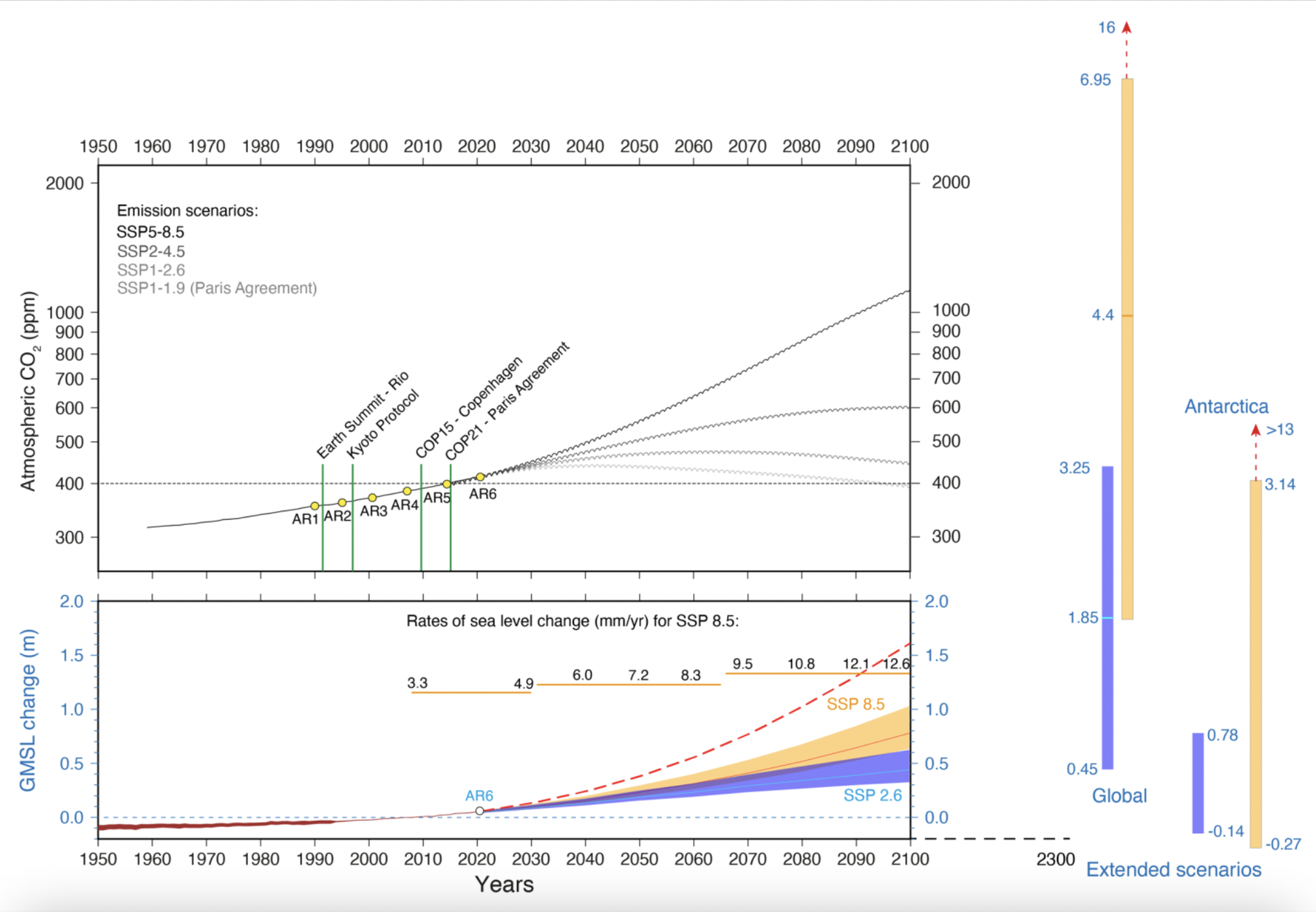
Detailed caption: Click image to enlarge. The evolution of atmospheric CO2 concentration (in parts per million, ppm) observed at Mauna Loa Observatory in Hawaii from about 1960 until 2020 (smooth black curve) is shown (top), along with projected CO2 concentrations (rippled gray curves) for various shared socioeconomic pathways (SSPs) until 2100. Years when key UN Framework Convention on Climate Change COPs were held are shown together with the IPCC Assessment Report publication years. Observed (brown) global mean sea level change (GMSL) from 1950 to 2020 (Frederikse et al., 2020) is shown (bottom) relative to the 1994-2015 baseline, along with projected changes for SSP 1-2.6 (blue) and SSP 5-8.5 (orange) and the 83rd percentile for the low-confidence SSP 5-8.5 (dashed red line) from IPCC AR6. The uncertainties indicated correspond to the 17th and 83rd percentiles for each SSP. Rates of sea level change (in mm/year) for SSP 8.5 until 2100 are also shown. The projected ranges (17th to 83rd percentiles) of GMSL change and the Antarctic contribution until 2300 AD (extended scenarios) from IPCC AR6 are plotted (right) for SSP 1-2.6 (blue) and SSP 5-8.5 (orange) pathways and for the 83rd percentile of the low-confidence scenario SSP 5-8.5 (dashed red arrows, accounting for instability processes in Antarctic ice sheet projections).
Another single model that accounts for both MICI and MISI produced a higher estimate of 20-53 centimeters (13th-87th percentiles) for the likely range of the Antarctic contribution to GMSL rise by 2100 for the SSP 5-8.5 scenario (DeConto et al., 2021). Moreover, under this model scenario, marine-based sectors of the AIS cross a tipping point of runaway ice loss before 2100, committing the planet to sea level rise of as much as 2 metres by 2100 and 15 metres by 2300 (Golledge et al., 2015; DeConto et al., 2021).
If, however, the global emissions trajectory follows the lower-emission scenario SSP 1-2.6 (figure 1), which is consistent with the emission target set by the Paris climate agreement, then the Antarctic contribution will likely be significantly lower: 12-31 centimetres by 2100 (Edwards et al., 2021) and about 100 centimetres by 2300 (DeConto et al., 2021). Under this scenario, models indicate that most of the Antarctic ice shelves would be preserved even on multicentury timescales, substantially limiting ice loss to the ocean (Golledge et al., 2015; DeConto et al., 2021).
A GMSL rise of about 25 centimetres by 2060 may be unavoidable. But notwithstanding results from our most sophisticated models to date, our poor understanding of key melt rate-determining processes and our uncertain emission trajectory create deep uncertainty in probabilistic sea level projections beyond the mid-21st century (e.g., Edwards et al., 2021; DeConto et al., 2021), impeding efforts to plan for impending changes along shorelines. This uncertainty suggests, for example, that there is a 5% chance that the Antarctic contribution to GMSL rise could be as much as 145 centimetres by 2100 (Bamber et al., 2019).
A further complication to understanding Antarctic contributions to sea level rise is that melting ice sheets do not cause globally uniform changes. When melting ice flows into the ocean, it changes Earth’s gravitational field and rotational state, thus redistributing water in the ocean; in addition, the remaining ice exerts less pressure on the land below, causing the land to rise. This viscoelastic response of the solid Earth to ice loss, a process known as glacial isostatic adjustment, means that locations near a melting ice sheet experience less sea level rise than more distant locations, with deviations of up to 30% of the global mean.
Crucially, these processes also feed back into ice sheet dynamics (Whitehouse et al., 2019). Unlike most feedbacks affecting ice sheets, which amplify mass loss, glacial isostatic adjustment might help stabilise a retreating ice margin by creating subglacial pinning points on the seafloor. Understanding how glacial isostatic adjustment influences Antarctic ice sheet dynamics and how they affect patterns of regional sea level change are critical areas of inquiry for improving site-specific sea level projections.
An INSTANT solution to tackle uncertainty
To advance understanding of processes affecting ice melting and reduce the deep uncertainty in Antarctica’s contribution to past and future sea level change, SCAR, an international coordinating body for Antarctic science, launched the INSTANT program. The initiative is following a multidisciplinary Earth system approach combining studies of geology, geophysics, atmosphere and ocean sciences, and glaciology to investigate long-term to short-term interactions among the ocean, atmosphere, solid Earth, and AIS.
INSTANT will build upon high-impact research and networking capacity developed within previous SCAR strategic research programs, including Past Antarctic Ice Sheet dynamics, Solid Earth Responses and Influence on Cryospheric Evolution, and Antarctic Climate in the 21st Century. These programs began addressing a key priority that arose out of IPCC AR5 and is one of six priorities identified in SCAR’s first Antarctic and Southern Ocean Science Horizon Scan: to better understand how, where, and why ice sheets lose mass. Seven years on from when the Horizon Scan was concluded, great progress has been made on this priority (Florindo et al., 2021). But because of the long lead times needed to acquire critical field observations that help us better understand physical processes and to incorporate these observations into next-generation numerical models to improve projections, the most important work lies ahead in the coming decade.
The ambitious goals of INSTANT and its partner organisations (e.g. the World Climate Research Programme) call for large-scale scientific cooperation over the next 8 years focused on three main themes:
- Improving understanding of atmospheric and ocean forcing on ice sheet dynamics
- Elucidating how solid Earth processes and traits, such as glacial isostatic adjustment and the roughness and depth of the subglacial bedrock, affect ice sheet dynamics and regional to global nonuniform sea level change
- Integrating new scientific results to improve reconstructions and predictions of the AIS contribution to sea level change and communicating and applying these projections beyond the research community
Engaging scientists and stakeholders
INSTANT’s leadership is international and includes researchers from a range of career stages. Overall, the program already has more than 200 members from more than 40 different countries. The program will facilitate knowledge sharing and build synergic efforts to carry on multiple campaigns among these members by fostering multidisciplinary international research collaborations as well as by organising workshops, publications, and training schools.
This collective approach will provide opportunities to bridge existing professional networks, increase the use of data banks, and spark new ideas and collaborations among scientists in different communities, such as those collecting observational data and those developing predictive models, to address technical challenges and push scientific frontiers. With its demographic diversity, as well as the diversity of science it will facilitate, INSTANT also provides an ideal framework to train a new generation of Earth scientists capable of informing pressing societal needs to better anticipate and manage future sea level rise.
Scientific outcomes from INSTANT – especially updated and more accurate projections of the rates, magnitudes, uncertainties, and likely impacts of Antarctica’s contribution to global sea level rise – will be important to a wide range of stakeholder groups. Crucially, as emphasised in its science and implementation plan, INSTANT will bridge this science with efforts to craft policy related to sea level change and to assess the potential effectiveness of, and risks associated with, climate change mitigation pathways and adaptation options. This effort will involve communication to and engagement with Earth system scientists, social scientists, practitioners, decision-makers, planners, and the public.
The outcomes of this communication and engagement will help guide adaptation approaches around the world to avoid the worst impacts of sea level rise as shorelines shift and communities, infrastructure, and ecosystems are inundated.
Florence Colleoni, National Institute of Oceanography and Applied Geophysics, Trieste, Italy. Tim Naish, Antarctic Research Center, Victoria University, Wellington, New Zealand. Robert DeConto, department of geosciences, University of Massachusetts Amherst. Laura De Santis, National Institute of Oceanography and Applied Geophysics, Trieste, Italy. Pippa L. Whitehouse, department of geography, Durham University, UK.
This article was originally published by Eos and has been republished here under a Creative Commons license.