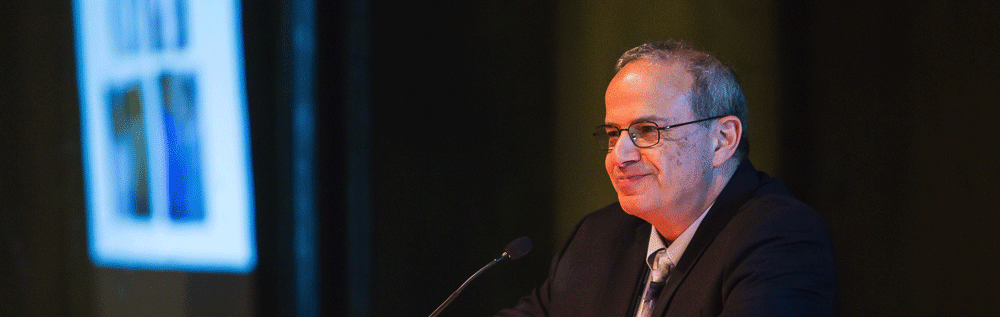
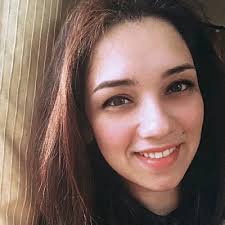
Sandhya Ramesh is a science writer with a focus on…
Bengaluru: At an event organised by the International Centre for Theoretical Sciences, Sandhya Ramesh caught up with Nathan Seiberg for an interview for The Wire. Seiberg is a string theorist at the Institute for Advanced Studies, Princeton. His work includes fundamental contributions to the development of string theory and improving our understanding of quantum field theory. Among other honours, Seiberg received the prestigious Dirac Medal in 2016. The interview has been edited for clarity.
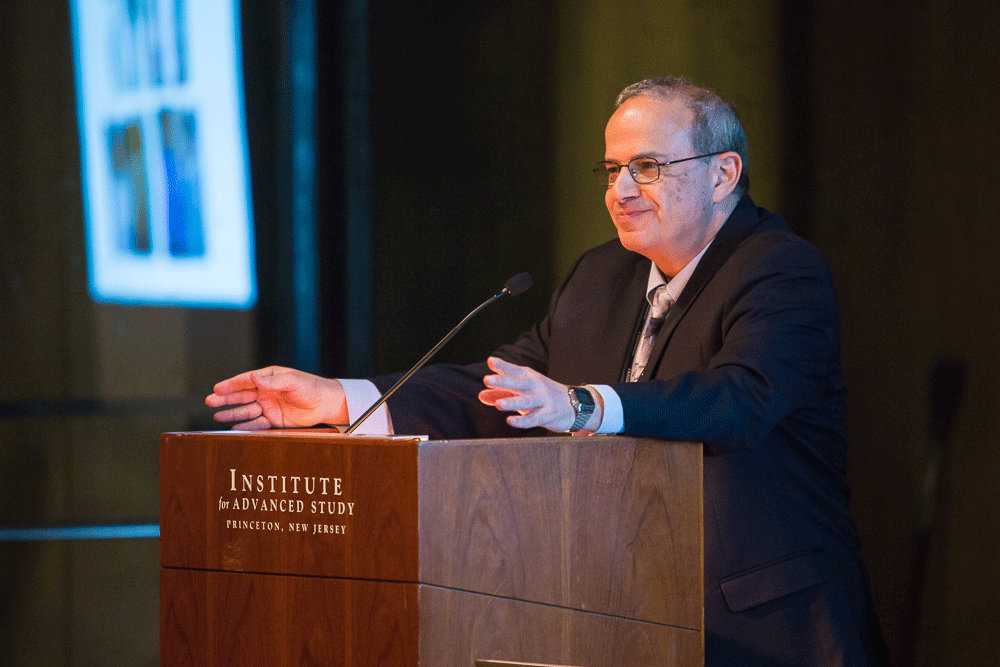
Sandhya Ramesh: How would you define string theory for a layperson?
Nathan Seiberg.: It’s a very ambitious attempt to find a complete theory of the universe, including all forces, all particles, and space and time. This is a long endeavour that has been going on for a long time and will continue to do so. It’s not something to be judged based on our success in the last quarter [century]. And the perspective on what it is and how to think about it has been changing over the years. I have no doubt it will continue to change, but I’m also confident that we’re making a lot of progress. It’s very exciting.
S.R.: What’s your opinion on reconciling the lack of any experimental evidence of string theory and the intense amount of theoretical work being done on it?
N.S.: It’s not reconciliation. There are two correct statements. One is that at the moment there is no experimental evidence. We don’t know when the verification will come or where it will come from. Maybe someone smart will figure it out next year, maybe it will take three years, maybe 300. We don’t know. The other thing is that there is some effort going into it. You can ask whether it is justified. And I can justify it on several fronts.
First, this is a very exciting intellectual effort and is as such worth pursuing. Second, it has given a lot of options to other branches of science, including condensed matter physics, ideas in astrophysics and cosmology, all the way to completely revolutionising ideas in pure mathematics. This means two different things: 1. This effort has already paid off, “paid off” by the standard criterion of having an impact on other things. 2. I think this is more important: Whenever you work on something and try to solve one problem, and you end up helping or solving many other problems, it is a sign that what you are doing is good.
Because historically this is the way it has worked. For example, Columbus tried to find India and ended up solving a much bigger problem. Other places like people working on quantum mechanics for the sake of understanding what matter is made of, and all modern technology including computers came out of it. This was the original intent. So when you work towards something with a long term goal and along the way you have all these options, a) the option has already been paid for, and b) it is a sign that it’s worth pursuing this work.
I don’t think there is anything to reconcile here because there is no conflict.
S.R.: When theoretical physicists try to explain ‘n’ dimensions, their typical example is ants walking on a wire. What is your favourite way to do this?
N.S.: I don’t have a favourite way (laughs). I use the same metaphor that you used, which is ants walking on a wire or a balloon. I don’t think anyone, including the most powerful mathematically minded people, can really visualise more dimensions. So it’s not that we can visualise and laypeople can’t. It has nothing to do with imagination. We have mathematical formulas that allow us to think about it. All these metaphors are only so good; they are never perfect. It’s a deficiency that we don’t have a better way of expressing and visualising. It’s a problem even for us. We just have to live with that. No one promised it would be easy.
S.R.: Do string theorists work on string theory itself or more on string-theory-inspired subjects? What do you think about this for the future?
N.S.: You found the right person. Of all the people who work on string theory here, I am very much interested in quantum field theory. A lot of that was motivated by string theory. A lot of work I do now has been a part of the work I’ve done on string theory, but a lot of the problems I solve today can be explained and answered without ever mentioning string theory. You can’t say this is strings and that isn’t. Different branches of the sciences are intrinsically connected. It is often the case that ideas in one branch influence other branches. So when we try to put people in boxes (“this person does condensed matter physics”), it becomes too narrow a view. In my understanding, especially here at ICTS, one of the visions is the unity of science. Different fields come together and people interact with each other, both to exchange ideas and for collaborations. It’s a very stimulating environment.
S.R.: Would you say the future lies in interdisciplinary work?
N.S.:I wouldn’t say ‘interdisciplinary’. There isn’t work being done on say string theory and climate science; they are too far apart. But there are neighbouring fields and in multiple such interfaces there are such interactions. Whenever it has happened, it has been very fruitful to both sides. We don’t know how the fields will evolve and which ideas will influence the future. I think it’s reasonable to expect that there will be such collaborative work and an institute that encourages it will be perfect.
S.R.: What does the future look like for particle colliders?
N.S.: I don’t know the politics of it. I think it’s a very important thing and there will be interest. It’s a lot of money. In the big scheme of things, it’s actually not a lot of money. It will all pay back. I can give you several examples, like how working on colliders paid off in unexpected ways – like the world wide web was invented at CERN and all the accelerators are now being used for research in medicine, biochemistry, etc. It’s not like we wasted money on the accelerator. It’s the opposite: we have got more out of it. We don’t know what the technology will give us in the future.
In addition, there is a very interesting fundamental question to be addressed. The flip side of that is something that I know nothing about the politics of getting someone to write such a big check. It’s very expensive and it is a long term commitment. I am always an optimist, so I hope it will somehow workout.
Another aspect is that it would take a long time to build. So even if someone puts all the necessary money today, they’re talking about projects that take five, 10, 15 years to complete. It’s a long time.
S.R.: That’s right, governments change.
N.S.: Even if governments change and the funding continues, it’s a challenge for the researchers who work on that time scale.
S.R.: I have a technical question. Could you describe some of the recent work on the relationship between gauge theories and global symmetries, especially with relation to condensed matter physics?
(Editor’s note: Gauge theories are a type of field theory that have been used to successfully model the behaviour of elementary particles like photons, electrons, quarks, etc. The Standard Model of particle physics is a gauge theory. Global symmetries are symmetries of nature that underlie conservation laws, such as those of energy and angular momentum. Condensed matter physics, as Seiberg put it, “is the study of materials and their properties”.)
N.S.: Okay, who gave you this question? (smiling)
S.R.: This is from my editor.
N.S.: Really? Wow, I’m impressed.
S.R.: I write about astronomy, he loves quantum and theoretical physics.
N.S.: Because this is right kind of question. The talk I’m giving here [at ICTS] on January 8 will be exactly on this point. It’s called ‘Symmetries, Dualities, and the Unity of Physics’. What I will talk about is precisely symmetry and condensed matter physics.
Symmetry has played a very important role in physics over the last few centuries. Physicists have been interested in symmetries and so have the lay people. The Taj Mahal is a very beautiful structure and one of the reasons it is so is, when you stand in front of it, you see this beautiful symmetric structure.
S.R.: Similarly with human faces as well…
N.S.: Yes, and also sometimes the face is beautiful when there is a slight imperfection and is not exactly symmetric.
I think it happened a few centuries ago that physicists got interested in symmetry and that influenced physics in many different places. The many different notions of symmetry that people use – like a lattice with periodic structure of atoms that repeats itself – that has a symmetry. You could rotate it and it looks the same. There are other sophisticated uses of symmetry, too. That is important in condensed matter physics, high-energy physics, string theory and particle physics. Over the last several years, it has become clear that many ideas of symmetries within two different fields are either the same or they complement each other. This is a place where there can be a lot of cross utilisation of different disciplines.
S.R.: Is the lecture on January 8 for lay people?
N.S.: It’s not. But it’s not as difficult as the following lectures. (laughs) It is at a collegiate level, which means an advanced undergraduate student in physics could understand it. I’m not recommending necessarily that you would come. I’m just surprised that the question was right on!
S.R.: We’ve found no experimental evidence of supersymmetry (SUSY) – but leaving that aside, what do you think SUSY theory people should be working on next?
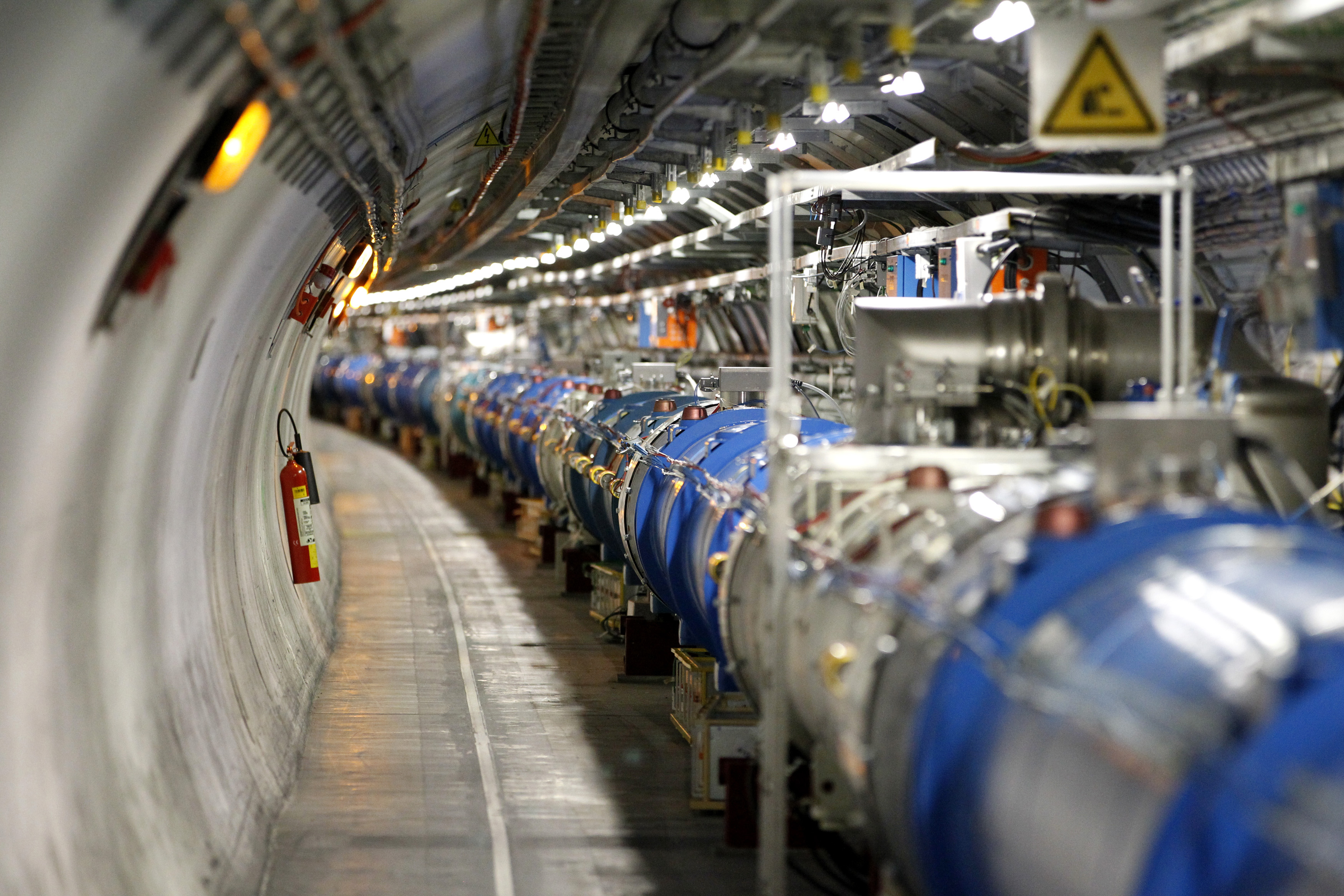
(Editor’s note: SUSY is a theory of ‘new physics’; it predicts the existence of certain fundamental particles that we haven’t observed yet. If these particles are found to exist, then SUSY is expected to be able to solve some open questions in modern physics.)
N.S.: I never tell people what to work on because my advice will end up being wrong. About SUSY itself, there are many different things when we say SUSY. One idea is that we will find SUSY particles at the Large Hadron Collider (LHC) but this hasn’t happened yet. So given that it hasn’t, I think the odds that it would happen in the near future are very small. It’s not a likely scenario. If you had asked me 10 or 20 years ago, I would have thought it was quite reasonable that they would find it. But they haven’t and that idea did not prove to be right. But people who worked on it should not be penalised for it because they just laid out possibilities and things that experimentalists would like to have.
But it turns out that the intellectual idea of SUSY has many other applications. It would have been nice had it been proven true, but even if it hasn’t been, the research has led to a lot of work and with a lot of impact in other places. For e.g., SUSY is absolutely central in the context of string theory. It led to a lot of the developments over the last 30 years or so, circled in one way or the other around it. The second thing is that it turns out that we can use models that we’ve played with, and sometimes, when the model is supersymmetric, we can actually solve it. So can’t solve models that we are really interested in, but these are viewed as cousins of the models we’re interested in. As such they’re interesting and they teach us a lot of things.
And the third avenue is applications in mathematics. It turns out that the ideas of SUSY that physicists have been playing with, these toy models that look “totally useless” for LHC physics, have had enormous impact on mathematics. For e.g., there was a conference or a school that just ended here this week and it was on Gromov-Witten invariance, which is a problem in mathematics. Most of the insight into it in the last few years actually came from physics and is heavily based on SUSY. This is like an intellectual structure that spreads hands in many directions and has applications in many different places.
One of them could have been the LHC. That has not materialised. It might or might not in the future, but at the moment it doesn’t look good. But it’s already given so much that it has already paid off. If we think of what physics and math would look like 50 or 100 years from today, independently of whether SUSY particles will or will not be discovered in colliders, it will play a crucial role. That’s completely obvious. So when you ask what SUSY people should work on, I would say just continue what they are doing.
I should also add that as theorists, even if we say it is a lot of effort, the cost of it is totally negligible. We are talking about, I don’t know, 300 to 500 people worldwide who do not need complicated equipment. So it’s not a lot of money. So when you say there’s been a lot of effort, it’s not that much. It’s a lot of money for you and me if we had to write a check, but for the government it isn’t a lot of money. And the impact has been huge.
S.R.: In your opinion, is M-theory the best contender for a ‘theory of everything’?
N.S.: I don’t have another one! It’s a contender without another contenders. Let’s leave it at that.
S.R.: Your thoughts on theoretical physics research being done in India v. that elsewhere?
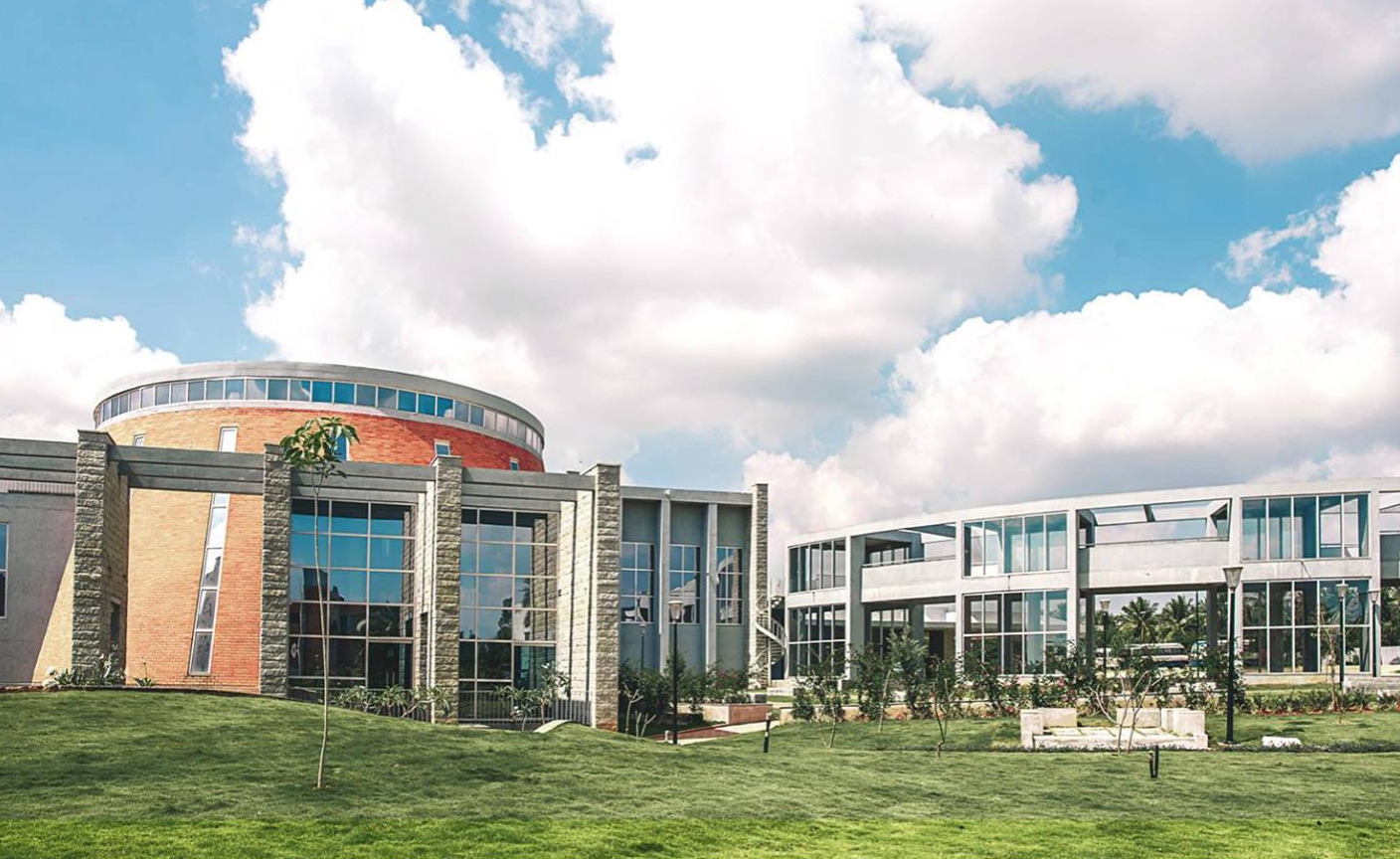
N.S.: Absolutely fantastic. Indian science is one of the best in the world in general – especially the effort in string theory and the circle of ideas from here are some of the best in the world. Indians are among global leaders in the field, like Ashoke Sen, Spenta Wadia, Sunil Mukhi, Rajesh Gopakumar, Shiraz Minwalla… I forget who else. These are people who wrote fundamental papers that changed the direction of the field. They could get jobs anywhere in the world, in the most prestigious of places, and they chose to come back to India because they felt that was important and I think India should be proud of these people. Home institutions should be proud of the fact that they are there. They educate the next generation of researchers and bring them to the same level of cutting edge. I come from the US and Indians are always in high demand when they come for postdocs or training. It’s really amazing that so many good people come out of here.
S.R.: Do you see a difference when you interact with the public here against those in other places?
N.S.: (laughs) I don’t interact with the public much. I talked with you today, and I gave a public talk a few years ago. That’s all I did.
S.R.: You should do more!
N.S.: I am not good at it.
S.R.: My opinion is that thanks to hyperconnectivity, conversations around science have really picked up and there is a lot of curiosity among the public.
N.S.: You guys and the popular science articles you write definitely plays a crucial role, too.
S.R.: Yes! And the social media is also a big help. Scientists are very accessible and it feels that the gap between scientists and public is being bridged.
N.S.: That’s good.
S.R.: I have another question from my editor. Do you think naturalness is a good or sensible guiding principle? Do you think it will remain useful or relevant as a guiding principle in the future?
(Editor’s note: There are some constants in theories of physics that describe the strength with which fundamental forces act relative to each other. They are called coupling constants, denoted by an alpha (‘ɑ’). When these constants don’t have the same order of magnitude – such as 1.1 and 123.4 instead of 1.5 and 9.4 – then naturalness is said to have been violated. For example, if ɑ = 1 for the strong nuclear force, then for the gravitational force, ɑ = 0.000000000000000000000000000000000000001. This means that the force of gravity is 39 orders of magnitude weaker than the force that binds quarks to each other. By abiding by the principle of naturalness, physicists question why this disparity in forces’ strengths arises. Those disputing naturalness would say that the disparity needn’t have any deeper meaning.)
N.S.: Ooh, he did his homework! If you had asked me 10 years ago, I would have said “Sure!” But given that the LHC has not found anything yet, I am much less sure today. I think it’s time to go back and examine and why we were so sure earlier and where we went wrong. I don’t see where we went wrong. In a way, it’s a very interesting situation where we had a logical reasoning chain without rigorous proof which led to this conclusion and nature tells us that that’s probably not true. We should go back and check where we went wrong because that’s how science works. When we say we believe something could be true, this is much more solid than most arguments in everyday life. And yet we would like to confront it with real data. When the real data tells us that’s not how things work, we should go back and reexamine. Something will have to give.
There was an interesting reasoning that led to naturalness, and now we know it’s probably not true. I think many people have been thinking where we went wrong and where the weak link is in this reasoning. And that’s good because we didn’t know this 10 or 20 years ago and now we do. We have a new clue which will hopefully allow us to make progress.
In the future: I don’t know, I am less sure now, with very low probability. But I think something will have to give. If it does not, someone will have to explain where the reasoning went wrong.
S.R.: In the near future, which aspects of theoretical physics do you think will gain attention, and where do you think a lot of work will be done?

N.S.: I have no idea! (laughs) I think we’ll be surprised. I can tell you what is currently exciting and I won’t’ be shocked if it’s totally different five years later. If you had asked me 10 years ago “What do you think would be exciting in 10 years?”, I would have gotten it completely. An example is the Laser Interferometer Gravitational-wave Observatory (LIGO). Ten years ago, most would have said, “Okay, maybe they’ll [detect gravitational waves]. Who knows. Most likely they will not find anything.” But they measured it and it’s a massive success. It has completely changed how we do astronomy today. It was predicted to happen 10 years ago. Some people had the vision but the mainstream excitement was just so-so.
S.R.: Especially with so many consecutive results…
N.S.: That’s right, so this is an example. There have been several others over the last 20 years. What the arena would look like 10 or 15 years in the future, I have no idea. I think LIGO will continue to be exciting, and I think the circle of ideas around string theory and quantum field theory will continue to be exciting. And connections between that and condensed matter physics, too. But what will specifically be exciting is hard to predict.
One of the reasons science is exciting – and I’m old enough to have seen a few cycles – is that we are constantly surprised. Either by experiment or by theory. Some smart person has a breakthrough and that completely changes our view of things. Now given that the nature of the field is that we are surprised by theory or experiment and that’s why it’s exciting, it means it is very hard to predict. It’s like asking “What will you be surprised by?” If you can predict it, it means you can’t be surprised by it! I think we have centuries of excitement.
S.R.: Do you visit India often? How do you like it? Have you visited any other cities?
N.S.: It’s my third visit here. I really like it. I visited Delhi and Agra a few years ago. I came to Bangalore two years ago.
S.R.: The weather is great here.
N.S.: I came for the colleagues! I know these people very well and have known them for a long time. It’s wonderful.
S.R.: Is the string theory scientists’ network small?
N.S.: Yes, we all know each other and it’s very small and wonderful.
Editor’s notes by Vasudevan Mukunth.
Sandhya Ramesh is a science writer focusing on astronomy and earth science.