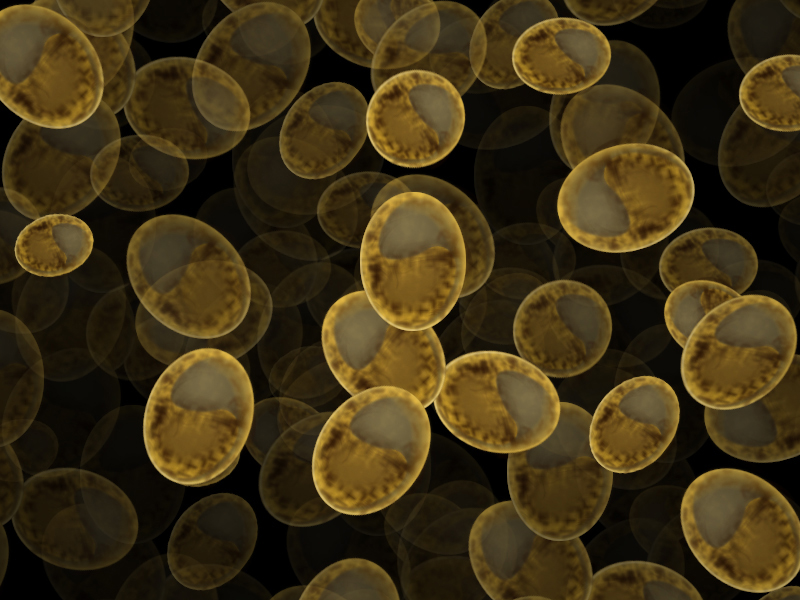
As a society reaping the benefits of all the great scientific discoveries coming from model organisms, it is imperative to continue to support such creative inquiry in as many systems as possible.

“Anything found to be true of E. coli must also be true of elephants.”
– Jacques Monod
Every year, in October, the Nobel prizes in the sciences, literature, peace and economics are announced. While for scientists it is a major story to be discussed or criticised, it is at best a minor news item for the most publications around the world. The irony here is obvious since Alfred Nobel, while endowing the prize, wanted to recognise individuals who “confer the greatest benefit on mankind”, and this is something that most prizes have accomplished – at least in the sciences.
This year was true to form by recognising superb, transformative discoveries that most people hadn’t heard about. The physics prize recognised work in topological phase transitions, which is something most people didn’t want to know about (even after the prize’s announcement) and yet may enable the greatest future applications in electronics and material science. Similarly, the chemistry prize recognised scientists who paved the path to make molecular machines, tiny groups of particles whose motion can be controlled when energy is provided and which make nanotechnology a reality. The prize in physiology and medicine recognised the discovery of the process of autophagy in cells, which has been one of the most exciting fields in cell biology for the past decade but which resulted in furious Googling by the public the day after the announcement.
We live in the best of times and the worst of times. And there hasn’t been a time in history before this with such a furious pace of discovery; at the same time, while we live in a Star Wars civilisation, most of us remain ignorant about these amazing times.
This sadly results not just in public apathy for science but also an under-appreciation for how amazing discoveries can come from seemingly unlikely or irrelevant systems. This year’s Nobel in physiology or medicine illustrates such an example. It was awarded for explaining the process of autophagy, where starved cells start eating themselves from within. The cells systematically degrade the larger bits and pieces within themselves in order to adapt and survive. These studies of autophagy are now considered classics in cell biology. Understanding the process of autophagy is pivotal for understanding the most important human diseases of our times, including cancer and neurodegeneration.
But here’s the kicker: most of the pioneering, conceptual breakthroughs in autophagy came not from studying human cells but from studying brewer’s yeast. And this Nobel recognition was hardly the first for brewer’s yeast – it was simply one in a long series, which started with understanding how cells managed to multiply and grow (a.k.a. the cell-cycle). All of these transformed our understanding of many diseases, most notably cancer. Other life-forms that have led to famous insights about the human body include other microbes, fruit-flies, nematode worms, frog eggs, rats and mice, etc. These are the model organisms that have transformed our understanding of biology.
The clichéd definition of a “model organism”, according to Wikipedia, is “a non-human species that is extensively studied to understand particular biological phenomena, with the expectation that discoveries made in the organism model will provide insight into the workings of other organisms.” But there is more to them and their history in science than this definition. Many model organisms came about due to a combination of serendipity, innate curiosity of influential individual scientists – and the ability of a model to be easily studied for a specific process. As our understanding of evolutionary biology expanded over the past century, we realised that findings from one organism could reveal very general principles applicable to many (or sometimes all) organisms.
A classic, storied case is that of the discovery of the concepts of genetic inheritance. Gregor Mendel was a Bavarian monk who, when a young novice in the monastery, wasn’t a particularly good one. He had entered the monastery because he wanted an education and the monastery provided a free one, which was perfect for someone with little means. But he had grown up gardening and beekeeping and was very good at both. Mendel was curious to know about sexual reproduction and inheritance but his abbot wasn’t too keen on a novice studying animal sex (with sheep), so Mendel was limited to studying the plants he enjoyed cultivating in the monastery’s garden.
He cultivated and studied a lot of different plants. It just so happened that the flowers of the sweat pea (Pisum sativum) had some features that made them good models for genetics. You can grow them easily, you can make pure sweat pea strains and, more importantly, it is possible to control their pollination. This is not true for all plants (especially many that both self- and cross-pollinate, something we appreciate now). Thanks to this bit of luck, Mendel worked out what the progeny of two crossed peas would look like. And all of this might have died with Mendel, who only presented his work (in German) to a local agricultural society; it was unknown to the famous scientific societies of Europe.
But a few years after his death, his work was rediscovered by two prominent scientists of the time, Hugo de Vries (who coined the term ‘genes’) and Carl Correns, both botanists working in famed institutes and cities and who were part of major scientific societies. They were working independently to understanding the laws of inheritance and defining the rules of genetics. They were influenced by the work of Charles Darwin, Alfred Russell Wallace and others in England. Both of them were fluent in German and therefore, when they stumbled upon Mendel’s work, were able to understand it.
They immediately recognised the implications of his work for the general rules of inheritance and acknowledged it in their theories of inheritance. But it was the neatness of Mendel’s observations, which depended so much on his model pea plant, which helped them complete and validate their ideas. So the type of model (and a bit of chance) helped discover one of the great, universal principles of genetics!
Model organisms continued to yield such major concepts. Thomas Hunt Morgan was another giant in the field of genetics and he did his work with the fruit fly. Morgan showed that genes are found on chromosomes and that they were the mechanical and functional units of heredity. He built his ideas on those of de Vries, Correns and others. Morgan wanted to understand heredity and mutation but since peas and other models had many difficulties, Morgan looked for another model. The fruit fly was a possibility because it reproduced fast and was ridiculously cheap to grow.
But what Morgan and his team at the “fly room” realised was that there were traits – or phenotypes – that were easy to follow. They used the colour of fly eyes (red or white) to understand inherited traits from parent to offspring and figured out that genes are arranged on chromosomes, like beads on a necklace, and that genes can be linked – where linked genes on the same chromosome will be inherited together. Their breathtaking work showed how useful the fly model was.
This has now created a legacy of using the fly to study everything from how organs develop to how disease processes start. With the molecular and genomics revolutions, we realised that many genes were in common between humans and flies. This has resulted in a host of discoveries, from understanding how some genes control smell, vision, motion, to how some genes control obesity or ageing or neuronal function. The easily manipulated fruit fly continues to provide scientists with some of our most fundamental discoveries.
We’ll end with the brewer’s yeast, an unlikely model for general concepts in biology. Yeast, more so than fruit flies (which eat yeast), are extremely easy to grow and humans have been doing that to make bread, beer and wine for millennia. But the reason they are useful models is because they – like all mammals (and us!) – belong to a glade called eukaryotes. Eukaryotes are different from bacteria because they have cells where the genetic material (our genes and chromosomes) are enclosed inside a nucleus and they also have other little specialised organelles inside them doing different jobs. So we can learn fundamental concepts of how eukaryotic cells work using the simplicity of yeast.
One of the first Nobel Prizes for work done in yeast was given to Lee Hartwell (with his colleague Paul Nurse, studying a different type of yeast, and Tim Hunt, who was studying sea urchins). This was for explaining the cell cycle, the processes by which cells replicate their DNA and then divide. The beauty of brewer’s yeast is that it divides by budding into an offspring cell. This allowed Hartwell and others to look at stages in the process of division. The other advantage is that it is easy to create mutants and screen for genes responsible for a phenomenon.
So yeast researchers have paved our understanding of how cells divide, how organelles are made inside cells, how chromosomes are maintained, how genes are controlled and how some proteins can cause neurodegenration and other diseases. All these studies have helped us move towards understanding human disease. And these are just a few examples of how model organisms have transformed our understanding of biology, life and human conditions. We’ve hardly touched upon the enormous understanding of biology coming from lab mice, the champions among model organisms.
So, coming back full circle, it is disheartening to see how little awareness the greater public has about model organisms and the need to support them. Anyone using model organisms in their research encounters mildly incredulous statements from friends and colleagues who express disbelief that such research is funded when not directly studying a human disease. This of course is the norm. Here are the words of former US vice-presidential nominee, former Alaska governor, and full time talking-head Sarah Palin:
You’ve heard about some of these pet projects, they really don’t make a whole lot of sense and sometimes these dollars go to projects that have little or nothing to do with the public good. Things like fruit fly research in Paris, France. I kid you not.
If you’ve read this far, you’ll agree that this statement is outrageous. Yet, it barely raised a public storm because, as far as popular perception goes, there really isn’t any popular perception on model organisms. Palin’s words are the mainstream.
As a society reaping the benefits of all the great scientific discoveries coming from model systems or organisms, it is imperative to continue to support such creative inquiry, driven by rigour and curiosity, in as many systems as possible. Thankfully, the best research agencies still recognise and support this, but this cannot be sustained (exclusively on taxpayer money or philanthropy) unless there is broad appreciation and support for such systems. I’ll end by badly paraphrasing the lyrics from Nobel literature laureate Bob Dylan‘s ‘Hurricane’:
But then they took him to the jailhouse
Where they try to turn a man into a mouse…
Don’t put the model systems and organisms of science in a prison cell
‘cos they truly are the champion of the world.
Sunil Laxman is a scientist at the Institute for Stem Cell Biology and Regenerative Medicine, where his research group studies how cells function and communicate with each other. He has a keen interest in the history and process of science and how science influences society.