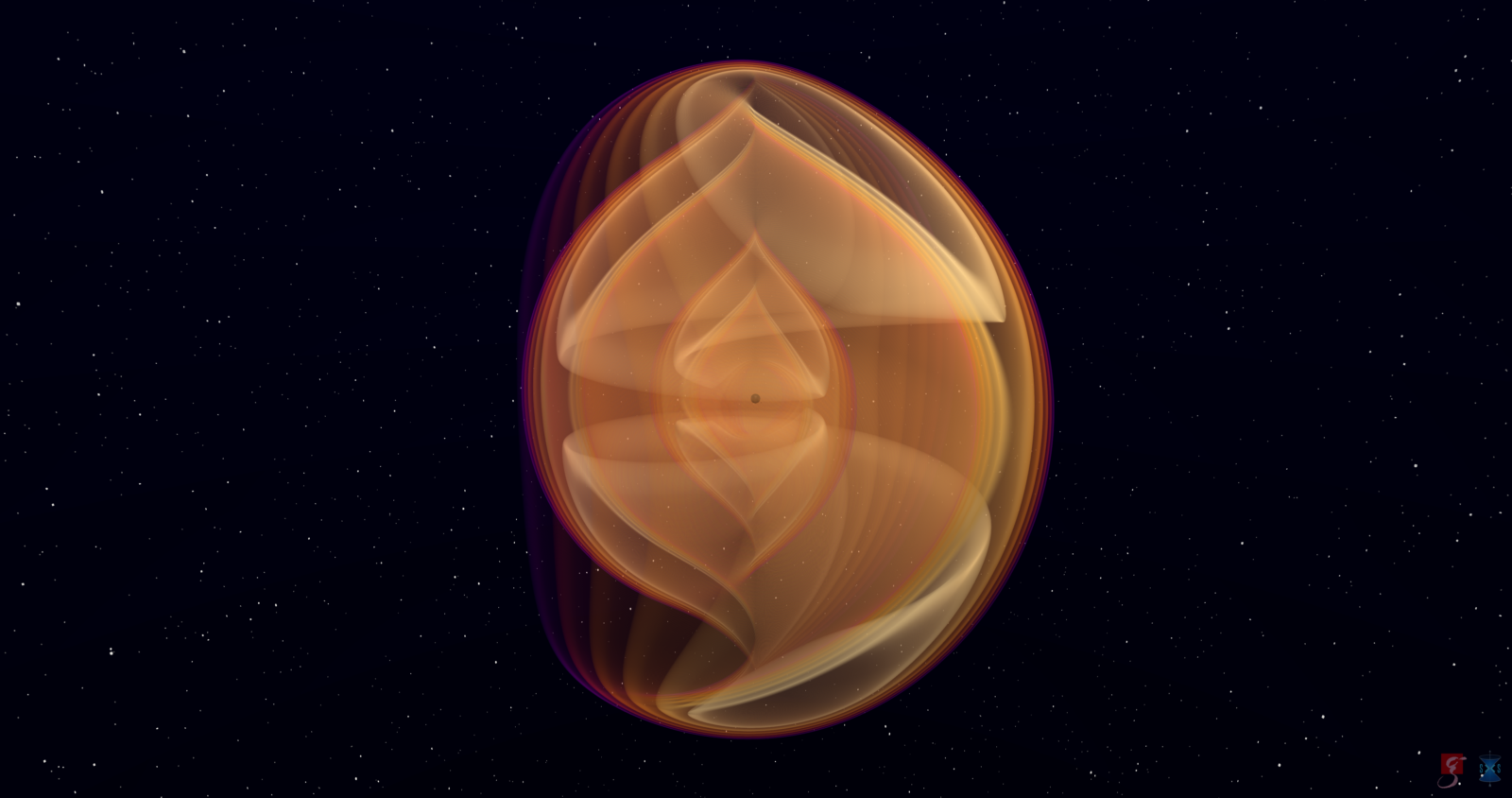
A visualisation of the merger of two black holes emitting gravitational waves. Source: N. Fischer, S. Ossokine, H. Pfeiffer, A. Buonanno (Max Planck Institute for Gravitational Physics), SXS Collaboration
- For more than a century, the theory of general relativity has passed every experimental challenge thrown at it, and is today one of the cornerstones of modern physics.
- While it has got many predictions right, we still don’t know if general relativity is the correct underlying theory of gravity – or if there could be some things it gets wrong.
- The scientists operating three gravitational-wave detectors, including the LIGOs in the US, used the largest trove of data to date to answer this question.
A rare manuscript detailing a crucial calculation from Albert Einstein’s theory of general relativity was recently auctioned off by Christie’s for $13 million [footnote]Rs 98.6 crore[/footnote]. This was testament to the theory’s continued hold on our collective imagination, scientifically and sociologically, more than a century after it was published.
General relativity has replaced the traditional interpretation of gravity as a force akin to the electric and magnetic forces with a more geometric picture – in which the fabric of the universe, called spacetime, interacts with its matter-energy content, with gravity arising as a consequence of this interaction.
For more than a century, this theory has passed every experimental challenge thrown at it, and stands today as one of the cornerstones of modern physics, the dominant theory shaping our understanding of the universe.
Birth of gravitational-wave astronomy
Before 2015, the only area where the theory’s relevance hadn’t been confronted was regions of spacetime that are highly curved and fast-changing. These are called the dynamical strong-field regimes of gravity. General relativity predicts that such regimes will be produced when compact objects like black holes come together and merge, ‘releasing’ information about the merger through ripples in the fabric of spacetime in the form of gravitational waves.
For the better part of the last three decades, two experiments in the US and one in Europe have worked together in pursuit of these fascinating predictions of general relativity: black holes and gravitational waves. In September 2015, they observed the first ripples from the merger of two black holes, and opened a new window into the universe called gravitational-wave astronomy.
The American experiments, or detectors, are the Laser Interferometer Gravitational-wave Observatories (LIGOs) in Washington and Louisiana states. They are exact copies of each other. The detector in Europe is called Virgo and is located in Italy.
The era of bulk detections
At the time of the first gravitational wave observation, the primary challenge was the problem of detection itself. But over time, the sensitivities of the LIGO and Virgo instruments have increased. Today, six years and three cycles of operation later, they have detected 90 gravitational wave signals. In November 2021, the LIGO-Virgo-Kagra collaborations (LVK) – Kagra is an upcoming detector in Japan – revealed a new haul of detections that included black holes of “all shapes and sizes”.
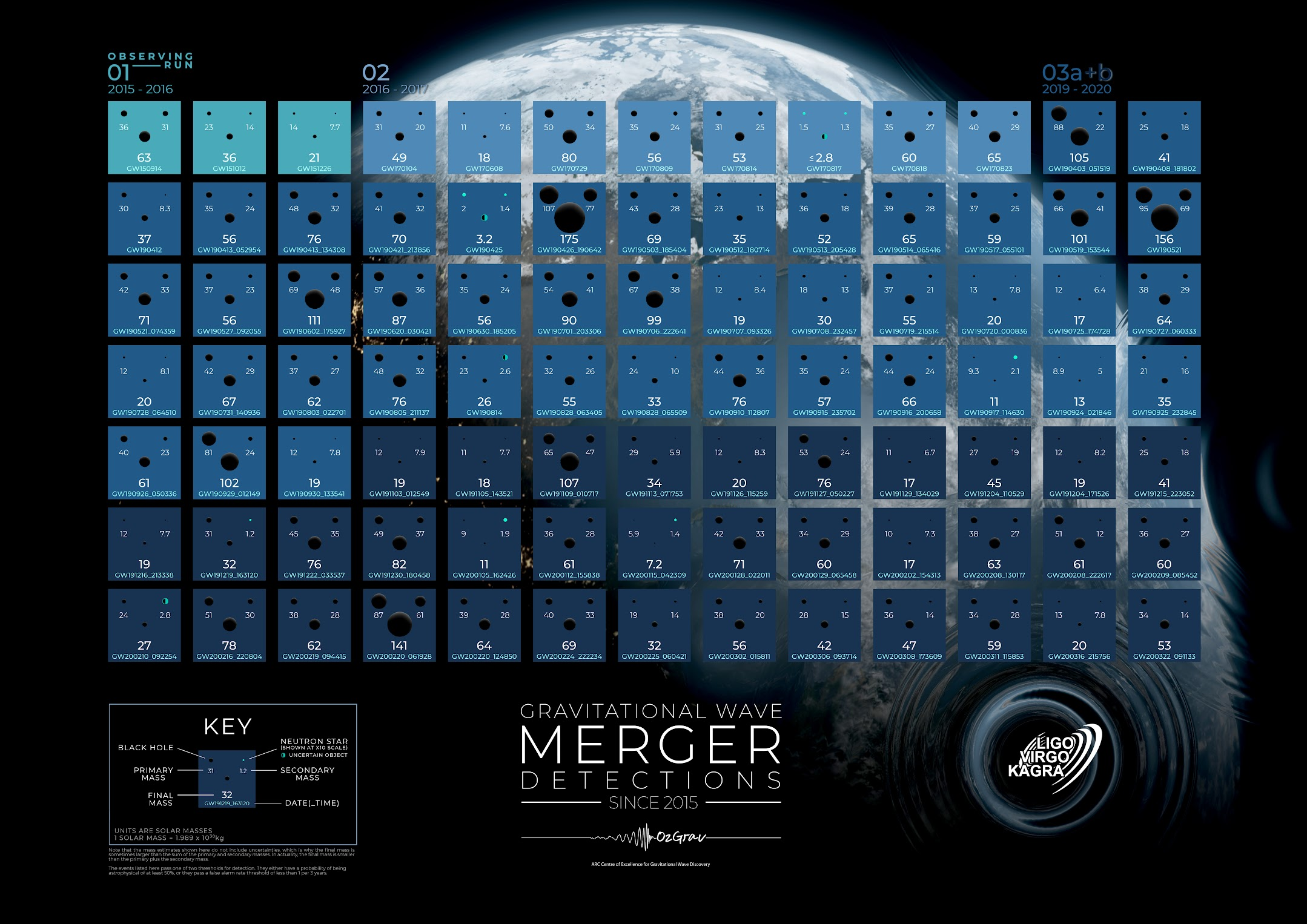
The instruments these days detect almost one new gravitational wave signal every week, and this pace is expected to increase in the coming years. So the focus of the field is slowly shifting from the problem of detection to their use in answering important questions about our universe – questions related to astrophysics, cosmology and particle physics.
Together with the catalogue of detections described above, the LVK collaborations, or just ‘LVK’, published two companion papers: one investigating how black holes could be distributed through the universe and another that explored the history of the expansion of the universe.
General relativity and gravitational waves
However, this carefully constructed pipeline of gravitational wave-data analysis, which starts with a detection and ends with making statements about the observable universe, is built on an important central assumption: that general relativity is the correct underlying theory of gravity. What if this is not the case?
Well, it would cast doubt on all our scientific findings thus far, starting with our acceptance of general relativity’s ability to accurately describe a gravitational wave signal and its measurable properties.
This is why whenever LVK release news of a gravitational-wave detection and any science done with them, their scientists simultaneously attempt to verify the robustness of the assumption that made it all possible: that general relativity is a valid theory of gravity. (The author is one of the scientists working with LIGO.) In this context, along with the scientific papers, LVK published a companion paper on December 14, 2021, and which attempted to answer one question:
Does Einstein’s theory of gravity hold up to the latest LVK observations?
The short answer is ‘yes’.
How are gravitational waves produced?
According to general relativity, when two black holes spiral in towards each other and merge, the resulting gravitational-wave signal – or general relativity waveform – has a characteristic profile that, as time passes, increases in frequency and amplitude until they peak. After the merger, the newly formed combined object slowly settles down into a single spinning, stable black hole, and the waveform rings down in a way resembling the sound waves from a bell or a tuning fork just after it has been struck.
Video: A visualisation of black holes moving around one another, one following a red trajectory, the other green. The arrows on each black hole indicate the axis about which they are spinning. As time passes, the panel (bottom) traces the waveform – the predicted gravitational-wave signal emitted by the merger of these two black holes.
These waveforms are routinely used to detect or analyse gravitational waves. And in the new paper, LVK scientists conduct tests to verify every aspect of these waveform templates.
When the two black holes are well-separated and moving relatively slowly, Isaac Newton’s theory of gravity can explain their dynamics quite well. But as they spiral in towards each other, they start moving faster, and physicists need to make a series of corrections to the Newtonian picture. These changes together form part of the post-Newtonian approximation, and are based on parameters defined by general relativity.
The black holes are also expected to spin, and a spinning black hole is deformed in the same way a rotating Earth is bulged at the equator and flattened at its poles. This ‘deformation’ leaves an imprint on the gravitational-wave signal, and the imprints can be described by other parameters in the post-Newtonian approximation.
Third, the combined black hole’s ringdown phase is expected to be made up of gravitational waves whose frequencies and decay times are specified by general relativity.
In their tests, LVK scientists relaxed these assumptions and allowed the parameters, frequencies and decay times to vary freely, instead of being fixed to those predicted by the theory of general relativity. This approach allowed the scientists (including this author) to check if the freely varying numbers, based just on the data collected, settled down to or near values predicted by general relativity.
At the end of the tests, the researchers measured how well the observed values and the predicted values matched. This helped them calculate the extent to which the observed values deviated from the theory. If there were significant deviations, it could have been an indication that general relativity is not a complete theory of gravity.
With their paper, LVK have confirmed that a gravitational wave signal can be described by a general relativity waveform to an unprecedented level of accuracy. Indeed, the results describe the strongest constraints placed on these waveforms (specifically, their deviation from theory) to date.
Also read: A Black Hole Paradox Where Relativity and Quantum Physics Meet
How do gravitational waves get to us?
Once two black holes have merged, they release gravitational waves through the spacetime continuum that travel at the speed of light. And just like photons, the carriers of light, the theoretically predicted carriers of gravity, called gravitons, are expected to have no mass. The LVK paper tested this hypothesis and improved on previously published results by up to twice the precision.
As they move out, gravitational waves are also expected to bend the surrounding spacetime. These bendings are called the polarisations of a gravitational wave. And general relativity allows gravitational waves to have only two kinds of polarisation: plus and cross.
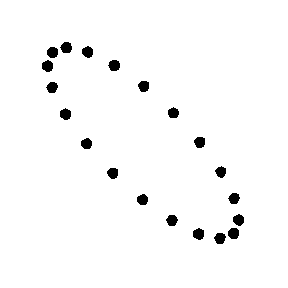
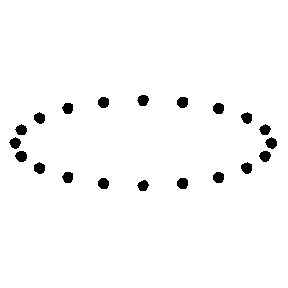
The two kinds of polarisation in general relativity – cross (left) and plus – as a gravitational wave passes into or out of the screen. Images: Public domain
A more general theory of gravity is expected to allow up to six kinds of polarisation. Using three gravitational-wave detectors – the two LIGOs plus Virgo – LVK found a way to set a partial limit on the beyond-general-relativity polarisation content of the observed signals. They have found that the data is consistent with the presence of just the two kinds of polarisation predicted by general relativity.
Search for impostors
According to theory, there could be some objects in the universe that mimic the behaviour of black holes but aren’t really black holes. One way to tell these mimics apart is using the ringdown part of a gravitational-wave signal.
Like the sound from a bell that has been struck, the waves from a black hole that is ringing down are expected to die down after some time. This is because every black hole has a characteristic surface around it called the event horizon. Any object (including light) or information behind the event horizon can’t escape into the universe. But black-hole mimics are predicted to have surfaces that can reflect some or all information back into the universe. These reflections can show up as echoes in the ringdown signal.
A search for such echoes in the observed gravitational-wave signals yielded nothing – implying that the source of the signals is consistent with rotating black holes in general relativity.
Consistency checks
The LVK paper ends with two consistency tests. The first checks if different portions of the predicted general-relativity waveform are self-consistent. That is, it checked if different portions of the same waveform yielded consistent information about its source.
The second test assumed that the data at the detector was a simple sum of a gravitational wave signal and detector noise. So if the general-relativity waveform is an accurate description of the underlying signal and is subtracted from the data, whatever is left behind should match our understanding of detector noise.
Like the rest of the analyses mentioned in the paper, LVK didn’t report any behaviour from these consistency checks that general relativity couldn’t explain.
In sum, the LVK paper outlines a battery of tests to validate our understanding of a gravitational-wave signal from mergers of black holes, using the theory of general relativity. And the tests, each one stringent, yielded no evidence that the gravitational waves our detectors have observed violate our theoretical predictions.
And by combining information from multiple gravitational wave observations, LVK has also been able to greatly reduce the uncertainty in their measurements, yielding the strongest bounds yet on possible deviations from the predictions of general relativity.
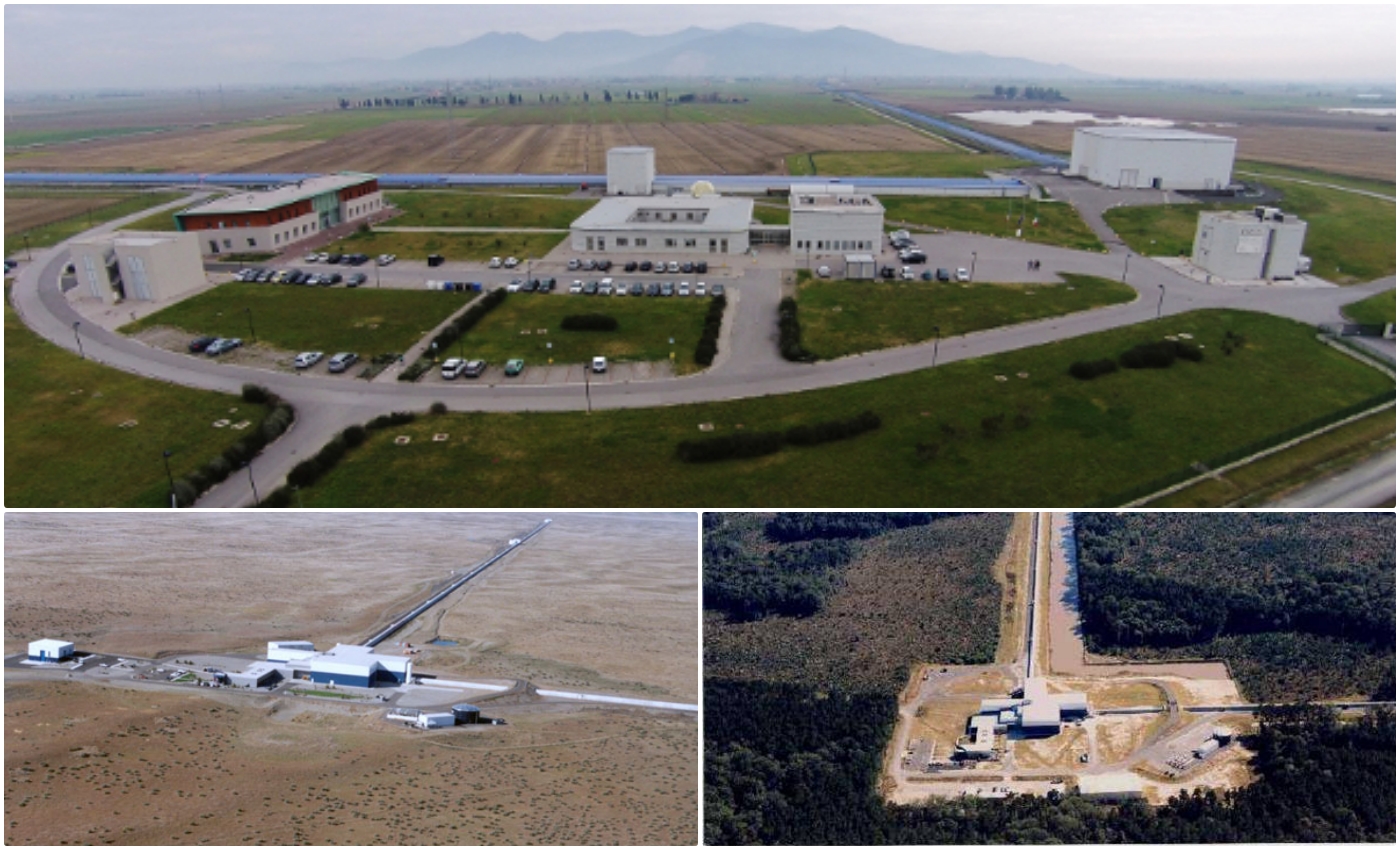
Towards a grand unified theory
One of the outstanding challenges of modern physics is the pending reconciliation between general relativity and quantum physics. We need to change one or both theories to come up with a single unified theory of the way subatomic forces shape the universe.
In the coming years, with the addition of new gravitational-wave detectors around the world, including LIGO-India in Maharashtra, and improvements to existing detectors, gravitational-wave detections are expected to become more frequent while simultaneously allowing us greater access to the observable universe.
At that point, we can realistically expect the new window of gravitational-wave astronomy to provide fresh perspectives on this old problem of a ‘Grand Unified Theory’. And for now, even if general relativity is not a correct theory of gravity, it’s surely not wrong by much.
Abhirup Ghosh is a junior scientist and a member of the Astrophysical and Cosmology Relativity group at the Max Planck Institute for Gravitational Physics (Albert Einstein Institute), Potsdam, Germany.