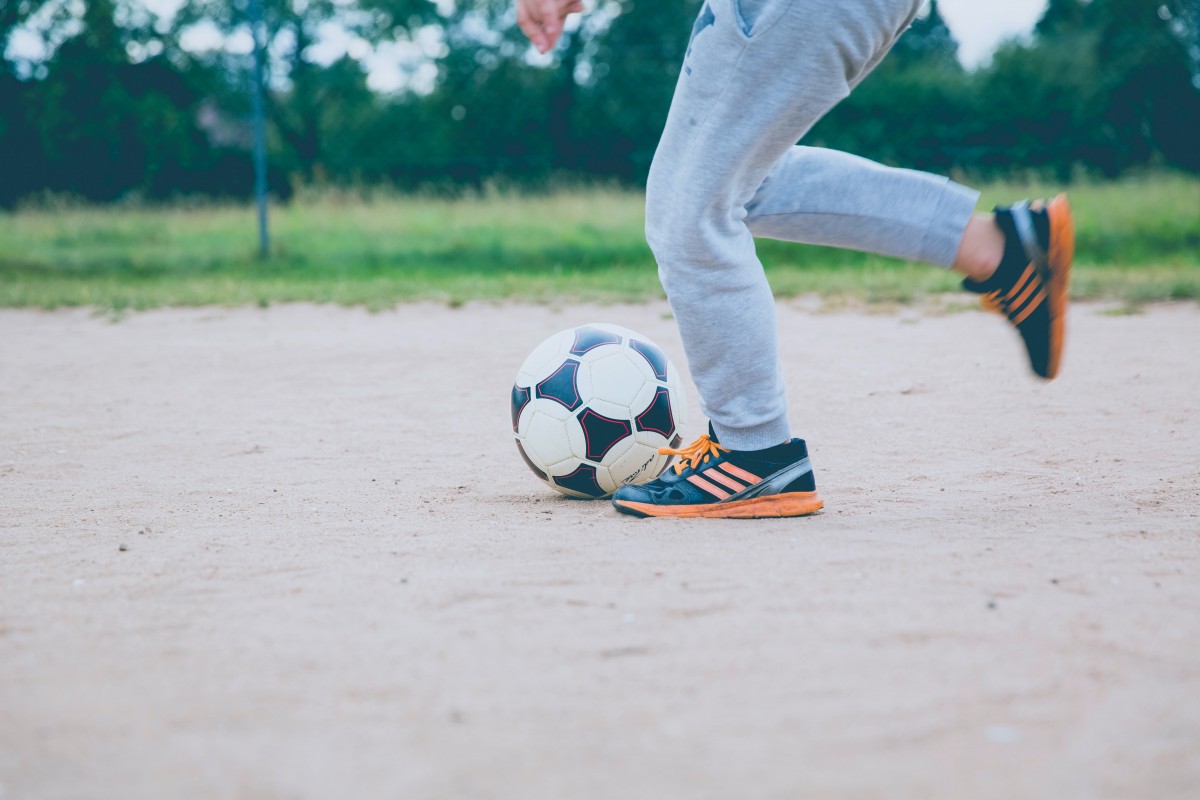
When you push on a swing that your friend is sitting on, you expect it to move forward. In physics parlance, this is called the law of conservation of momentum. The expectation that the swing will move forward is so obvious that it is, in fact, mundane. This is exactly why physicists were startled when they shined a laser on electrons and some of them moved backward.
Light carries momentum, so when it strikes the surface of a metal, according to Jared Strait of the National Institute for Standards and Technology, Maryland, it exchanges some of its momentum with electrons that are free to move on the surface. The electrons are pushed in a particular direction; if the incident light is at an angle, the net push on many electrons creates an electric current. This is called the photon drag effect.
For current to be produced along a long, thin strip of metal, light has to be able to push the electrons along the length of the metal. The momentum carried by light is in the direction it travels in, so shining light vertically onto the metal won’t create a current. There has to be a horizontal component, and the more horizontal the incident light is, the bigger the current.
Strait and his colleagues wanted to use the current to calculate the light’s momentum – a simple experiment. But they weren’t prepared for the results. “We did not expect any of this,” Strait told The Wire. “It’s surprising that we could discover something in such a simple experiment.”
The team illuminated a thin gold film with a laser at different angles. As they made the incident light more horizontal, they saw that the current increased – as expected. However, the occasional backward push was inexplicable.
The researchers repeated the experiment in different ways, sometimes changing the polarisation of light and sometimes keeping the setup in a vacuum. They found that only one form of polarisation, called p polarisation, caused the current to be in the forward direction as expected if the setup was also operated in air. For all other combinations of polarisation and air/vacuum, there was a backward current.
“The result seems fundamental,” Ashok Mohapatra, a professor of quantum optics at the National Institute of Science Education and Research, Bhubaneswar, told The Wire. This isn’t surprising. Physicists are supposed to have fully understood simple interactions like light pushing on electrons. If there are unexpected results here, it implies they could have missed an elementary part of the description.
Mohapatra said he couldn’t make sense of the backward current. When you strike a single atom that has been trapped on its own with photons, the results are simple, he said. If the atom is pushed forward, it will move forward.
One possible explanation for why things change depends on “what’s outside”, Strait said. He and his teammates believe different molecules present in the air attach to the surface of the metal and could explain the backward push.
“The difference between the vacuum and air states is the population of stuff on the metal,” Strait said. As a vacuum is created, more of these molecules detach, resulting in a different kind of surface at the molecular level than what existed when air was present.
They saw that if they started from a state with air present and slowly sucked it out, the direction of the current decreased, and eventually flipped. This gradual and continuous change suggests there is definitely an effect caused by air on light-metal interactions.
Also read: Under a Microscope, a Bizarre Electronic Dance Comes Into Better Focus
Such a relationship could explain the backward current but the physicists also think it could have applications for technologies that use metallic surfaces. For example, according to Strait, changes in the strength of a current could be used to measure how even a surface is at the level of a single molecule.
But on the theory front, Strait admits that even though multiple experiments point to the same thing, the result is quite surprising, and that it might take some time for people to fully accept it. One of the team’s key discoveries is the signal from the backward current: “Part of getting this paper published was convincing the referees and the editor that we didn’t make some silly sign error.”
Now that the paper has been published, physicists will need to find a way to make sense of the current in terms of what they know about how light reflects from a metal. And if this seems like a fundamental question, it’s probably because it is. “This seems like the sort of question that would have been pondered and settled a century ago,” Strait mused.
Sagnik Ghosh is a physics PhD student at Brandeis University, Boston. He has been on leave for the past year.