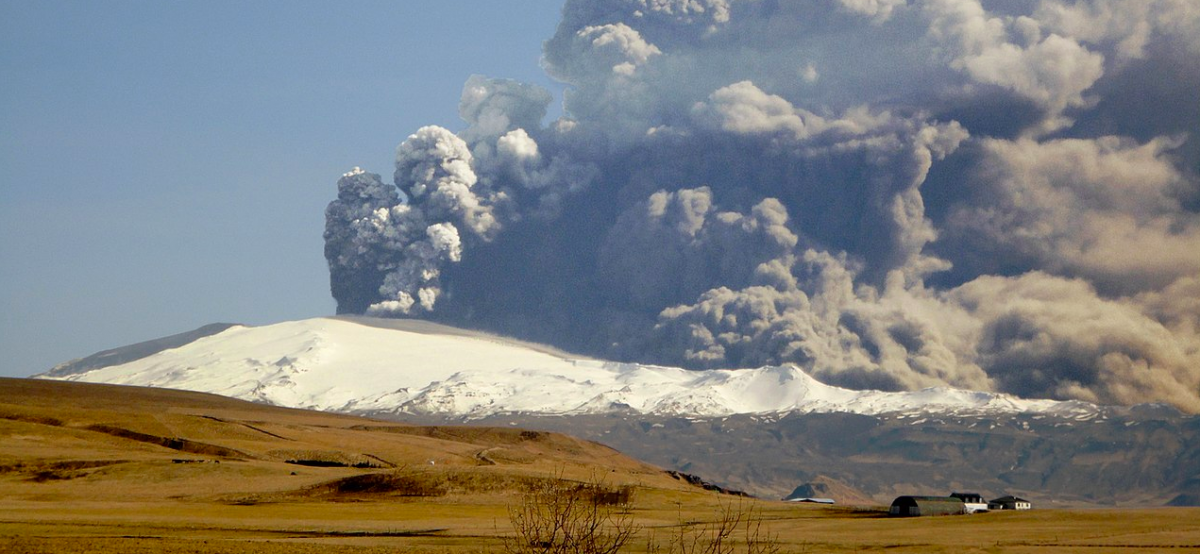
Ronak Gupta is pursuing a PhD in fluid mechanics at…
Volcanoes are agents of mass change and mass destruction. They have been responsible for extinction events in the past even as they maintain the important global carbon cycle.
For most of us, a volcanic eruption conjures the image of a mountain spewing hot lava, but that isn’t the most dangerous thing about it. In fact, you’ve probably never heard of it: it’s called pyroclastic flow.
When a volcano erupts, along with magma, it releases hot gases, ash and other particles, together with enough force to detach boulders, and everything smaller, from the mountainsides. This concoction of volcanic release rushes down like an avalanche, a devilish curtain of smoke and stone hurtling towards the ground. You’ve probably seen videos of snow doing the same thing, but its volcanic counterpart – called pyroclastic flow – is deadlier.
Pyroclastic flows can reach speeds of an F1 car at full throttle and temperatures well above the boiling point of water.
In 1902, when Mount Pelée in the Caribbeans erupted, pyroclastic flows levelled the entire town of Saint-Pierre and killed almost 30,000 people.
They have been known to travel long distances, over land as well as water, without losing their destructive potential. How is this possible?
A paper published last month made a giant stride towards an answer. Since you can’t stick a speedometer in the path of an actual pyroclastic flow – it would be smashed to pieces plus such flows are hard to come by – researchers from the US and New Zealand did the next best thing. They created fake pyroclastic flow resembling the real thing, in a lab, and ran experiments on it.
Its secret? Pyroclastic currents are able to flow so rapidly because there is a thin layer of nearly frictionless air below them that acts like a lubricating cushion.
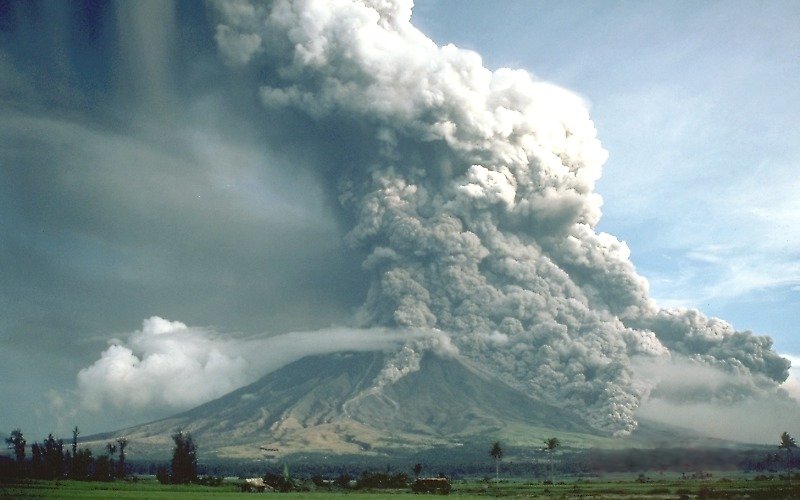
First, a giant vat heated a mixture of gas and particles obtained from an actual volcanic site. This energetic mixture was then dropped down a three-storey tower before it flowed onto a long slide and finally down a horizontal path. By changing the type of particles in the flow, the height of the drop, the slope of the slide and roughness of the path, the researchers could simulate different volcanic scenarios, and observe them with high-speed cameras.
This way, they found that a pyroclastic flow generally consisted of two parts: a lower, denser zone with solid particles and a high-speed gas cloud that floated above it. But when they zoomed in on the lower zone, they found that had two distinct layers of its own. All the solid particles were concentrated in the upper layer while the bottom one was much more sparse. This is where the magic happened.
When a fluid flows over a solid surface, many of its particles may slip over but most of them experience friction and slow down. In the same vein, the part of the pyroclastic flow closer to the ground slows down more than the fluid higher up. This is a blessing in disguise for the flow overall.
As the flow’s base slows down, the fluid’s pressure increases away from the base. As a result, gas from the top of the flow moves to the bottom, further diluting the suspension and allowing the current to cruise at higher speeds.
Gravity tries to play spoilsport – like it always does – by pulling the heavier particles down, but it is beaten for pace. The gas lubrication process packs the lowest layer with more gas faster than the heavier particles can sink.
Augmenting these observations with computer simulations, the researchers were able to substantiate their claim that air lubrication is the principal mechanism that ensures pyroclastic flows can move so fast.
The importance of studies like this is apparent in their applications, especially in disaster mitigation. While there might not be a completely effective way to protect people against a pyroclastic flow, knowing how it works can allow officials to assess risk more accurately and design better evacuation protocols.
A paper published almost 25 years ago reached a similar conclusion: that even a medium-scale eruption of Mount Vesuvius, Italy, could potentially destroy everything in a 7-km radius around it, including a population of one million (as of 1994).
But even if the experiments don’t go on to save any lives, the knowledge they have created is just as meaningful. As an editorial in the journal Nature on the pyroclastic flow paper concluded, “The combination of numerical models and laboratory experiments should be encouraged, not just when life and livelihood are at stake, but when greater scientific insight is the likely reward.”
Ronak Gupta is doing a PhD in fluid mechanics at the University of British Columbia, Vancouver.