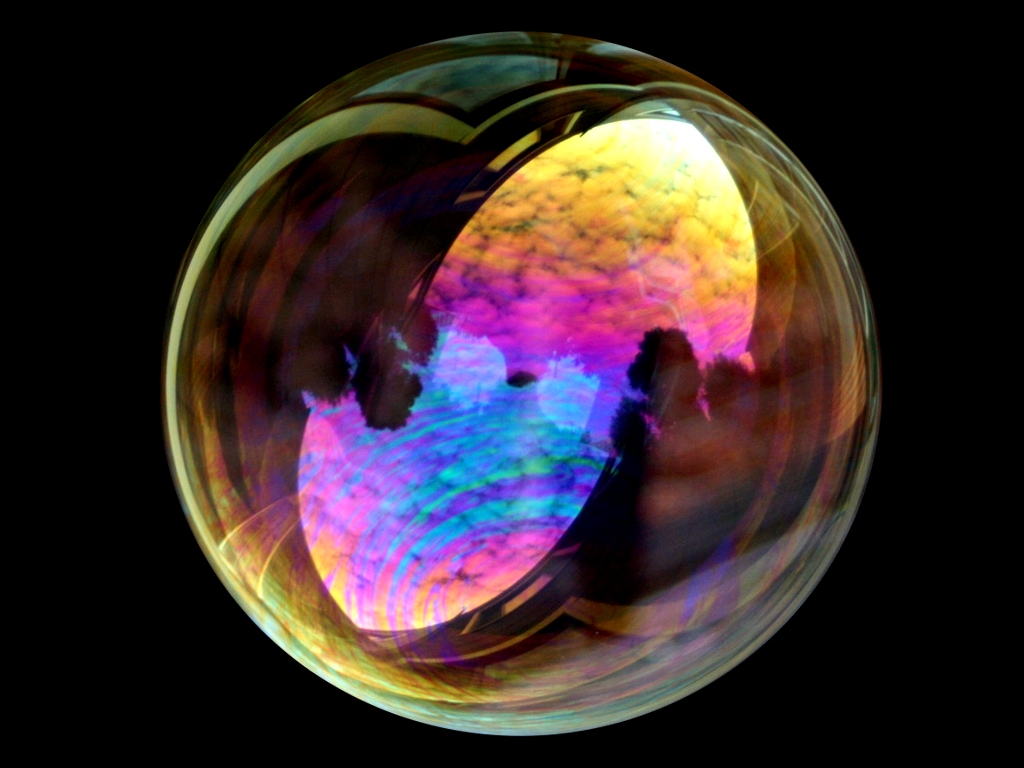
Thomas Young has been called “The Last Man Who Knew Everything.” In 1793, barely twenty years of age, he explained how our eyes focus upon objects at different distances, based partly on his own dissection of an ox’s eyes. A year later, on the strength of that work, Young was made a Fellow of the Royal Society, and in 1796 he became “doctor of physic, surgery, and midwifery.” When he was in his forties, Young helped Egyptologists decipher the Rosetta stone (which had inscriptions in three scripts: Greek, hieroglyphics, and something unknown).
And in between becoming a medical doctor, getting steeped in Egyptology, and even studying Indo-European languages, Young delivered one of the most intriguing lectures in the history of physics. The venue was the Royal Society of London, and the date, November 24, 1803. Young stood in front of that august audience, this time as a physicist describing a simple and elegant homespun experiment, which, in his mind, had unambiguously established the true nature of light and proved Newton wrong.
“The experiments I am about to relate … may be repeated with great ease, whenever the sun shines,” Young told the audience.
Whenever the sun shines. Young wasn’t overstating the simplicity of his experiment. “I made a small hole in a window- shutter, and covered it with a piece of thick paper, which I perforated with a fine needle,” he said. The pinhole let through a ray of light, a sunbeam. “I brought into the sunbeam a slip of card, about one-thirtieth of an inch in breadth, and observed its shadow, either on the wall, or on other cards held at different distances.”
If light is made of particles, Young’s “slip of card” would have cast a sharp shadow on the wall in front, because the card would have blocked some of the particles. And if so, Newton would have been proved right.
Also read: How the Rainbow Illuminates the Enduring Mystery of Physics
If, however, light is made of waves, as [Christiaan] Huygens claimed, then the card would have merely impeded the waves, like a rock impedes flowing water, and the wave would have gone around the card, taking two paths, one on either side of the card. The two paths of light would eventually recombine at the wall opposite the window shutter to create a characteristic pattern: a row of alternating bright and dark stripes. Such stripes, also known as interference fringes, are created when two waves overlap. Crucially, the central fringe would be bright, exactly where you’d expect a dark shadow if light were made of particles.
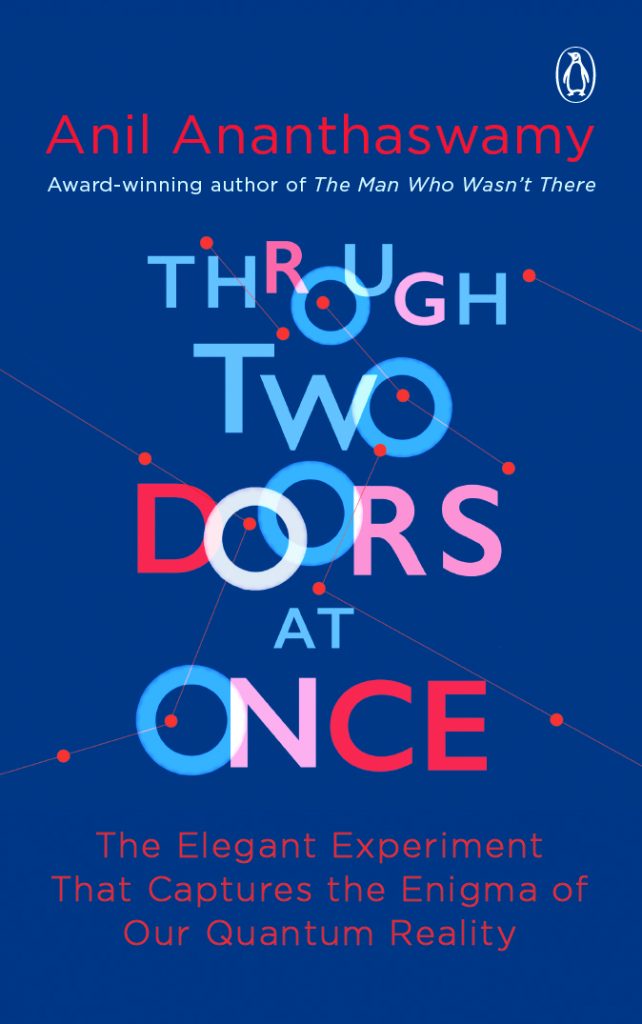
Through Two Doors At Once
Penguin Random House, 2018
We know about interference from our everyday experience of waves of water. Think of an ocean wave hitting two openings in a coastal breakwall. New waves emerge from each opening (a process called diffraction) and travel onward, where they overlap and interfere with each other. In regions where the crests of both waves arrive at the same time, there’s constructive interference and the water is at its highest (analogous to bright fringes of light); and in regions where the crest from one wave arrives at the same time as the trough of the other, the waves cancel each other out and there’s destructive interference (corresponding to dark fringes).
Young saw such optical interference fringes. Specifically, since he was working with sunlight, which contains light of all colours, he saw a central region that was flanked by fringes of colours. The central region, upon closer inspection, was seen to be made of light and dark fringes. The numbers of these fringes and their widths depended on how far away the pinhole in the window shutter was from the screen or wall. And the middle of the central region was always white (a bright fringe). He had shown that light is wavelike.
There must have been disbelief in the audience, for Young was going against Newton’s ideas. Even before Young’s lecture, articles written anonymously in the Edinburgh Review had been heavily critical of his work. The author, who turned out to be a barrister named Henry Brougham (he became Lord Chancellor of England in 1830), was scathing, calling Young’s work “destitute of every species of merit” and “the unmanly and unfruitful pleasure of a boyish and prurient imagination.”
It was anything but. Soon enough, Young’s ideas got further support from other physicists. His experiment led to what’s now called the double-slit experiment and was in fact the first formulation of it—the very same experiment whose virtues Feynman extolled during his lecture at Cornell. In the more standard double-slit experiment, Young’s sunbeam is replaced by a source of light. And instead of a “slip of card” placed in the sunbeam’s path to create two paths for the light, the double-slit experiment creates two paths of light by letting the light fall on an opaque barrier with two narrow slits or openings through which the light can pass. And on the screen on the far side, you see an interference pattern, essentially fringes similar to what Young saw on the wall opposite the window shutter (if the screen is a photographic plate, or a piece of glass coated with photosensitive material, then the image can be thought of as a film negative: dark regions will form where the film is being exposed to light). You don’t see just two strips tapering away, which you’d expect if light behaved as if it came in lumps. It’s behaving like a wave.
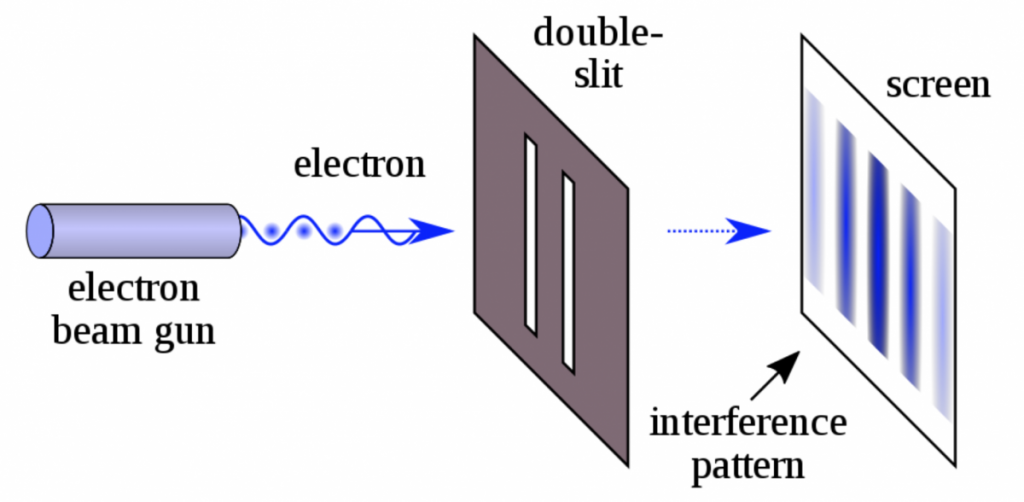
So, well before quantum physics was even a gleam in anyone’s eyes, Young had seemingly settled the debate between Newton and Huygens (despite skeptics who continued to favour Newton). Young came down in favour of Huygens’s light-is-a-wave idea. And so things stood until the quantum revolution.
The revolution began with bewildering discoveries in the early 1900s, including Albert Einstein’s 1905 assertion that light should be thought of as being made of particles, because it was the only way to explain a phenomenon known as the photoelectric effect (which interfering waves laser pointer helps us convert sunlight into electricity, giving us the technology of solar panels). These particles of light came to be called photons. For any given frequency or colour of light, a photon of light is the smallest unit of energy, and it cannot be divided any further: the light cannot come with any less energy than contained in one photon. Einstein’s argument is somewhat involved, but for now, if we accept the idea that there are certain situations in physics where you have to treat light as made of particles, then the double-slit experiment starts challenging our intuitive sense of reality.
Also read: Knabenphysik At 92: Remembering the Boys’ Club of Early Quantum Mechanics
Feynman spoke of the double-slit experiment as embodying the “central mystery” of quantum mechanics. To show why, he replaced the gun shooting bullets (or, in our case, grains of sand) with a source of electrons. Everyone in the 1960s knew that electrons came in lumps. They are one of the many types of elementary particles that make up the subatomic world, including photons. We’ll use photons instead of electrons. The fact that the experiment, its results, and its implications don’t change whether we are using photons, which are particles of light without any mass, or electrons, which are particles of matter with some mass, leads to its own set of mystifying questions. As Feynman said, both are screwy in the same way.
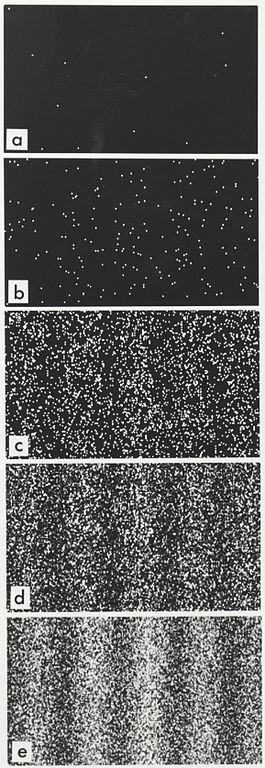
Here’s what happens if you use photons. Unlike what we got with particles of sand, you don’t get two bands of light on the screen. Instead, you get fringes, similar to the interference pattern that Young observed, suggesting that photons are behaving like waves. To get a sharply defined set of fringes, it’s best to use light of one colour. So the source can be, say, streaming out an intense beam of photons of red light that pass through the double slit.
When both slits are open, you get the interference pattern, suggesting that light (which we know now is made of particles) is going through both slits. But if you close one of the two slits (doesn’t matter which one), the interference pattern disappears, clearly suggesting that light is going through only one slit and there’s nothing for it to interfere with.
The experiment, however, really starts messing with our minds when we consider a source that emits one photon at a time. We’ll come to the ways in which physicists invented sources to do that. It wasn’t possible in 1964, when Feynman was giving his lecture. For now, let’s assume we have such a source in hand. If so, each photon distribution of electrons or photons has peaks and valleys. The peaks are places where more particles land, and the valleys are places where fewer or no particles land source of particles goes through the apparatus, and we make sure there’s only one photon passing through the setup at a time. The photon hits the photographic plate on the far side and creates a spot. If we let enough spots accumulate, our intuition says that these photons should act like grains of sand and line up behind each slit. There should be no interference pattern.
We’d be wrong. As it happens, even though each photon seems to be landing at some random position, fringes emerge when enough photons have made their mark on the photographic plate. Each photon makes a dark spot on the plate; places where the photons mostly land become dark stripes, and fringes build up over time.
This is somewhat curious. It’s clear that we can get an interference pattern when one wave interferes with another. But our photons are going through the apparatus one by one. There’s no interference between one photon and the next, or the first photon and the tenth, and so on. Each photon is on its own. Nonetheless, each photon is mostly landing on the photographic plate at those positions that eventually become regions of constructive interference and mostly avoiding those places that become regions of destructive interference. We get interference fringes. It’s as if each photon is exhibiting wavelike behaviour, as if it’s interfering with itself.
This is happening even though we create each photon as a particle, and detect it on a photographic plate as a particle: the results seem to suggest that between the creation and detection, each particle acts like a wave, and somehow goes through both slits simultaneously. How else do you explain the interference pattern?
Also read: The Graceful, and Graceless, Pursuits of Peace in the Quantum World
If that’s not mysterious enough, consider what happens if we try to find out which slit a photon goes through (our intuition, after all, says that it surely went through just one slit, not both). Say you have a mechanism for detecting the passage of a photon through one or the other slit without destroying the photon. If you do that, the interference pattern goes away (meaning the photon stops behaving like a wave and starts acting like a particle)—and you get a pattern that’s simply the sum of the “lumps” going through each slit. Stop trying to sneak a peek at the photon’s path and it goes back to behaving like a wave—the interference pattern reemerges.
There’s yet another way to appreciate this mystery. When you are not looking at the photons’ paths, individual photons almost never go to certain places on the photographic plate—the places that eventually become regions of destructive interference. But if you start monitoring their paths, they will go to the very locations that they otherwise shun. What’s going on?
The curious behaviour continues. If you were to fire grains of sand at the double slit, and if you knew everything about the initial conditions of each grain of sand (its initial velocity, the angle at which it leaves the sand gun, etc.), you can predict using Newton’s laws exactly where the grain of sand will end up on the screen opposite the double slit, taking into account any deflections due to the interaction with the slits. This is how physics is supposed to work. But you can’t do that with photons (or electrons, or anything quantum mechanical for that matter).
Even if you have all the information about a single photon as it leaves the source and goes toward the double slit, you can only calculate the probability of the photon landing on a certain part of the photographic plate. For example, the photon could land at any one of the many regions of constructive interference—but there’s no way to tell exactly where any particular photon will go. Nature, at its deepest, seems inherently nondeterministic. Or is it merely hiding its secrets, and we haven’t dug deep enough yet?
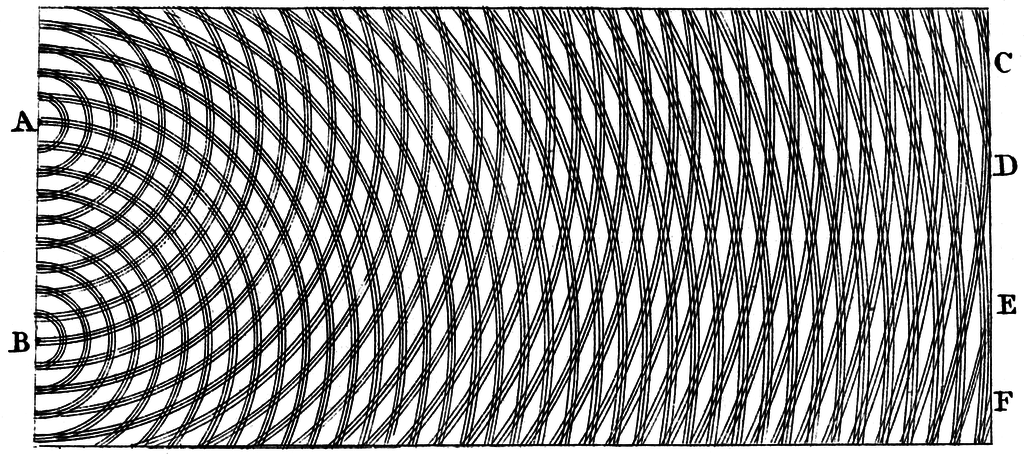
The questions pile up. Between the production of the photon and its eventual detection, both proofs of its particle nature, the photon ostensibly behaves like a wave if we choose not to look at which path it takes, and as a particle otherwise. Does the photon “know” we are looking at its wave nature or particle nature? If so, how? And can we fool the photon, say, by not revealing our hand until it has crossed the double slit as a wave, and only then choosing to see which slit it went through, thus examining its particle-like behaviour?
Maybe there is a simpler answer: that the photon is always a particle and always goes through one or the other slit. And something else, something that our standard theories don’t account for, goes through both slits to produce the wavelike behaviour. In that case, what is that something?
If it crossed your mind that human consciousness is somehow involved in causing the photon to behave one way or the other, you wouldn’t be alone in thinking so. As often happens when confronted with two mysteries (in this case the odd behaviour of the quantum world and the inexplicable nature of consciousness), it’s almost human nature to want to conflate the two.
It’d be twenty years on from Feynman’s lecture at Cornell that the double-slit experiment would be done using single photons. It was an example of how, from Young’s efforts in the early 1800s to modern versions, physicists continue to use the double-slit experiment to understand the nature of reality. The experiment hasn’t changed in its conceptual simplicity for more than two hundred years, but it has become technologically more and more sophisticated, as experimenters keep thinking of clever ways to trick nature into revealing its profoundest secrets.
Excerpted with permission from Through Two Doors At Once by Anil Ananthaswamy (Penguin Random House, June 2018).