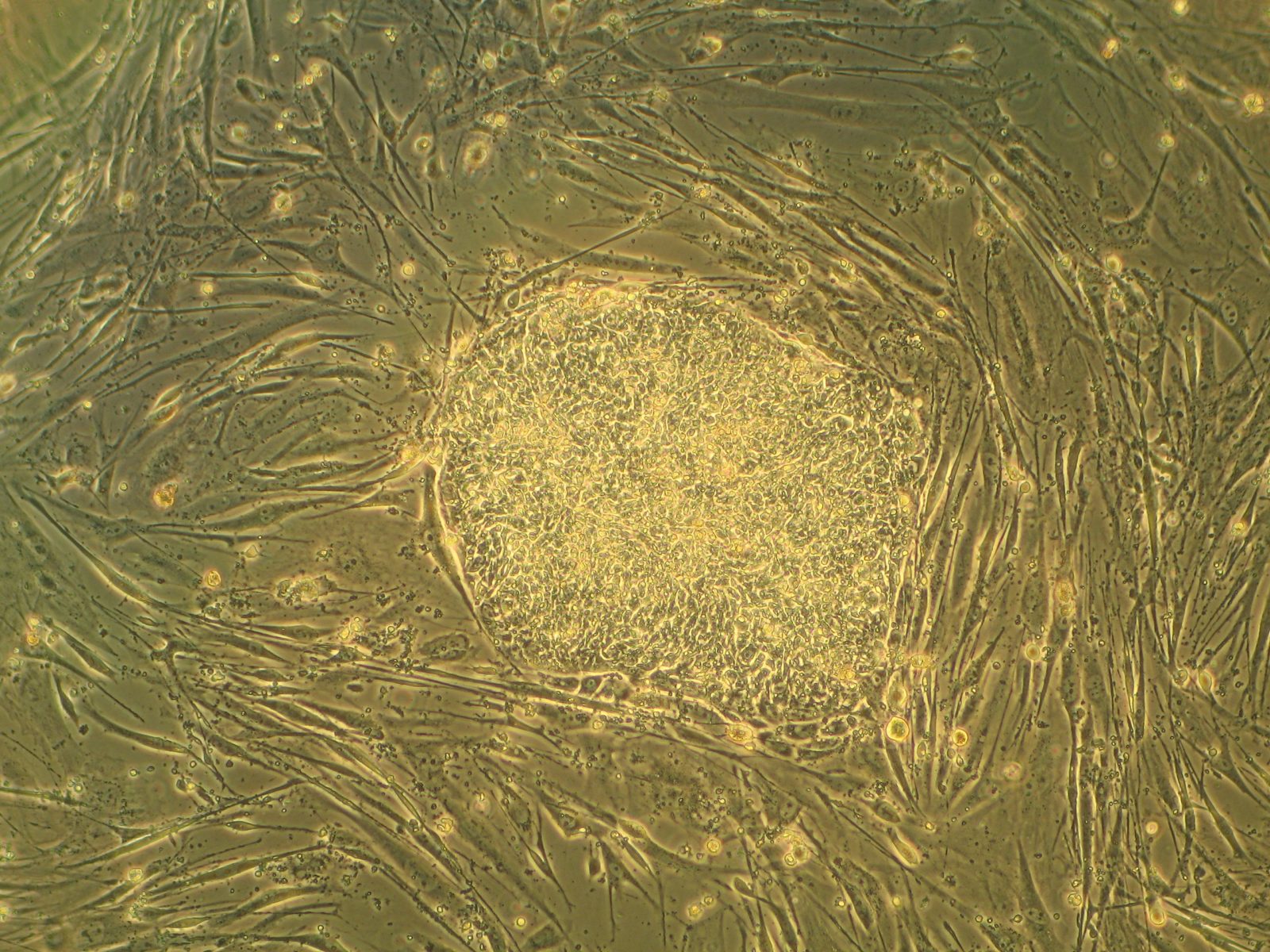
A colony of human embryonic stem cells (centre) from the H9 cell line. Image: Ryddragyn/Wikimedia Commons, public domain
- Stem cells are special cells that develop into different cell types in the human body, for example, nerve cells or muscle cells, making them highly desirable candidates for treating diseases.
- Scientists from the University of Allahabad identified the core genes that allow stem cells to exist in distinct functional states.
- Knowing the exact molecular mechanism behind these states paves the way for newer methods to culture stem cells and use them in therapeutic or biomedical applications.
Scientists from the University of Allahabad, Prayagraj, are closer to understanding how human stem cells work. They have identified the core genes that allow stem cells to exist in distinct functional states. Knowledge of the exact molecular mechanism behind these states paves the way for newer methods of culturing stem cells and using them for therapeutic or biomedical applications.
Stem cells are special cells that develop into different cell types in the human body, for example, nerve cells or muscle cells, making them highly desirable candidates for treating diseases. Stem cells are of two kinds : adult and embryonic stem cells (figure 1). Adult stem cells primarily develop into a particular cell type – for example, muscle tissue stem cells will only give rise to muscle cells. On the other hand, embryonic stem cells (ESCs), found in three to five-day-old embryos, are pluripotent; that is, they can grow into almost any type of cell in the adult body. However, owing to ethical issues, human embryonic stem cells are not used for laboratory experiments and therapeutic applications.
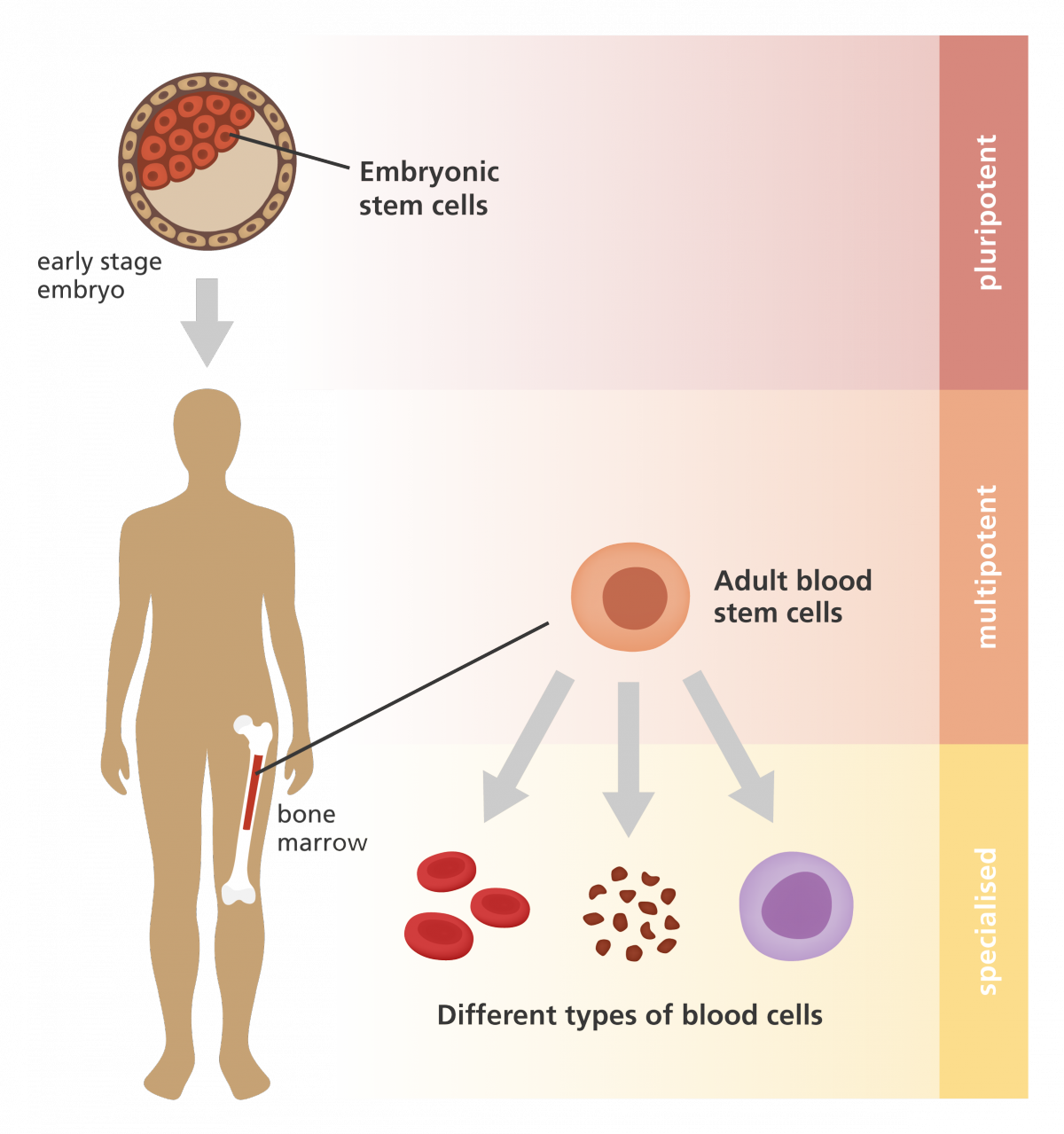
Interestingly, research has shown that cells of the body (e.g., skin cells, blood cells, etc.) can be altered in the laboratory to behave more like embryonic stem cells. These are called induced pluripotent stem cells (iPSCs) (figure 2).
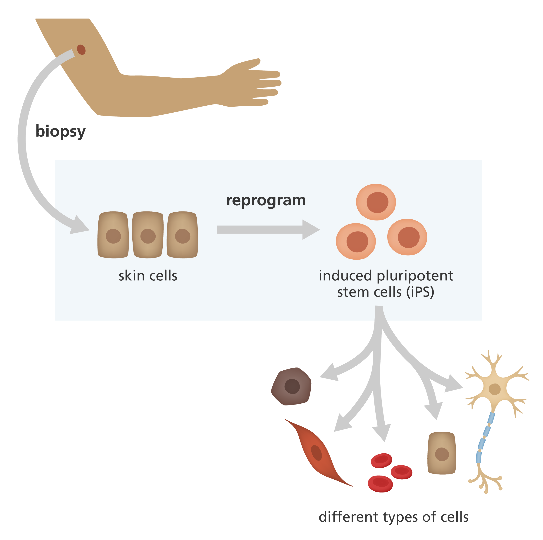
Pluripotent cells exist in two distinct functional states – naïve and primed. Naïve cells are unrestricted and grow into any cell type, whereas primed cells are inherently biased towards their cell type and are not as amenable as their naïve counterparts. Anup Som, principal investigator, University of Allahabad and corresponding author of this study, says, “iPSCs are an effective alternative to embryonic stem cells. However, they are most useful when equivalent to naïve cells. Hence, it is important to understand the mechanism behind the two states of human pluripotency.”
Rakhi Pal, chief technology scientist, Institute for Stem Cell Science and Regenerative Medicine (InStem), Bengaluru, who was not part of the study, adds, “Greater clarity on the role of naïve and primed pluripotent cells in the origin of diseases can lead to targeted therapies and more specific drugs.”
Som’s team gathered gene expression data from previously published studies on naïve and primed human ESCs. They then used a bioinformatics method called weighted gene co-expression network analysis (WGCNA) to study the correlation between different sets of genes. The application also helps to identify a gene or a set of genes that cause a particular disease.
Using WGCNA, the team hoped to identify groups of genes that work in tandem to execute a specific function – in this case, maintaining the stem cells in either the naïve or primed state. Two sets of genes stood out; the first set consisted of 4,791 genes with the most significant activity in the naïve state, while the second set comprised 5066 genes responsible for the primed state of pluripotency.
Their next step was to identify which of these genes code for transcription factors (proteins controlling the activity of a gene). The researchers compared their list of genes to a previously published catalogue of human transcription factors and found that 52 transcription factors were critical for the naïve state and 33 factors for the primed state. Thus, these 85 transcription factors can now be considered key to controlling the switch from naïve to the primed state of pluripotency.
Pal highlights the challenges associated with such a study, “In biological research, new data emerge as we speak. Integrating findings from various research groups worldwide can be tough, and cell culture largely depends on the prevailing conditions in different laboratories. Normalising all that data to reach a stage where one can compare the results is an uphill task.”
Som and his team have their eyes set on their next goal. He says, “In the current study, we focus only on the protein-coding genes. In future studies, we aim to incorporate the role of non-coding DNA (DNA that does not directly provide instructions for protein synthesis, but indirectly may help in regulation) in the maintenance of pluripotency”.
This article was first published by IndiaBioscience and has been republished here with permission.