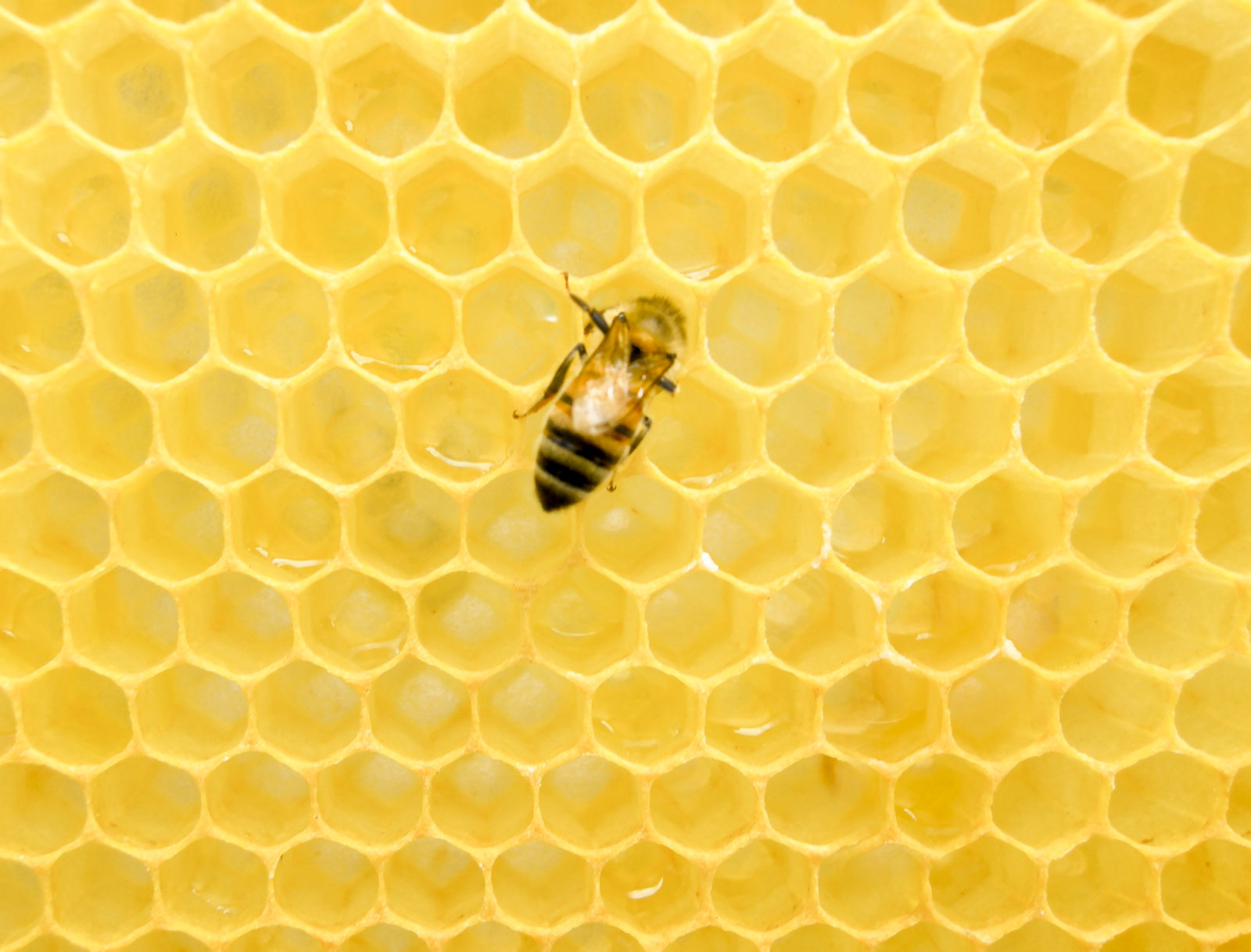
A portion of a honeycomb, showing the precisely constructed hexagonal cells; all but one bee have been removed. Photo: Matthew T. Rader/MatthewTRader.com, CC-BY-SA
- The honey bee nest is a masterpiece because of its engineering sophistication and mathematical precision.
- The complex structures and decisions of insect societies arise from what has come to be called swarm intelligence.
- The concept of stigmergy has become the organising principle to understand how simple, apparently uncoordinated activities of individual insects can build up to complex patterns.
In the grand Indian epic ‘The Mahabharata’, the evil king Duryodhana, instigated by his cunning uncle Shakuni, ordered his chief architect Purochana to build a ‘Lakshagriha‘ – a palace made of wax (or lac) in the forest of Varnavrat. His intention was to trap his rivals, the five Pandava brothers, and their mother Kunti here and to set fire to the palace at night, and kill them all.
Every time I hear this story, I feel a pang of anxiety for the honey bees who build for themselves architecturally magnificent palaces of wax and live in them in the tens of thousands, enacting the drama of their fascinating lives, venturing out only to pollinate our crops and orchards, thus contributing significantly to our food supply and making human civilisation as we know it possible.
If the weaver ant nest is a marvel because the adult ants somehow persuade their larvae to donate silk to build a communal nest, and the underground nests of fire ants and harvester ants are amazing because they build exquisitely functional nests in the darkness of the underground, the honey bee nest is a masterpiece because of its engineering sophistication and mathematical precision.
The honey bee comb is a sheet of wax with a regular back-to-back array of hexagonal cells. The base of each cell, which is shared by cells on both sides, consists of three rhombi (a rhombus has four sides with both pairs of opposite sides parallel and all sides the same length). It is widely appreciated that the honey bee comb is designed optimally. The hexagonal cells are better suited to rear cylindrical larvae as compared to triangles or squares. Circular cells might be better, but they leave too much empty space in between.
Unlike pentagons, heptagons or octagons, hexagons can be packed on a plane without gaps. Indeed, an array of hexagons maximizes space use and is most economical in the amount of wax used.
How and why do the honey bees make such an immaculately designed structure? These questions have intrigued and interested philosophers, scientists, engineers and mathematicians for centuries.
A mathematician’s verdict
In 1964, the Hungarian mathematician and geometer László Fejes Tóth (1915-) wrote a paper in the Bulletin of the American Mathematical Society, with the interesting title ‘What the Bees Know and What They Do Not Know’. With the abruptness and precision befitting a mathematician, the paper begins with the sentence, “In the first part of this paper, we construct a more economical honeycomb than that of the hive bees for any parameters involved in the problem.”
After describing the honey bee comb, Tóth asks, “Why do the bees build such a strange conformation?” Based on his calculations, he gives credit to the bees for choosing hexagonal cells, saying, “In this respect, the bees make a good choice, because of all convex plane-fillers of given area the regular hexagon has the least perimeter”. But in the matter of the cell bottoms, he says, “Instead of closing the bottom of a cell by three rhombi, as the bees do, it is always more efficient to use two hexagons and two rhombi.” I was a bit crestfallen, but he immediately says:
“We must admit that all this has no practical consequence. By building such cells the bees would save per cell less than 0.35% of the area of an opening (and a much smaller percentage of the surface area of a cell). On the other hand, the walls of the bee-cells have a non-negligible width which is, in addition, far from being uniform and even the openings of the bee-cells are far from being exactly regular. Under such conditions the above “saving” is quite illusory. Besides, the building style of the bees is definitely simpler than that described above. So we would fail in shaking someone’s conviction that the bees have a deep geometrical intuition.”
In the matter of “deep geometric intuition”, Tóth was probably referring to his fellow mathematician and geometer, Pappus of Alexandria from the fourth century, famous for the Pappus hexagon theorem in projective geometry. He had concluded that the honey bees “were endowed with ‘a certain geometrical forethought’”.
It’s not just honey bees, and it’s not just nest architecture. Social insects such as ants, bees and wasps make stunningly complex structures and decisions that defy our expectations about what their small brains are capable of. It seems implausible that their brains could achieve these feats with the kind of individual intelligence and cognition that we are familiar with. So the view that theirs is a qualitatively different kind of intelligence has come to be accepted.
The complex structures and decisions of insect societies arise from what has come to be called swarm intelligence. Individual members of insect societies lack our kind of intelligence and have no plan or blueprint. Instead, they follow simple local rules that appear to have no relation to the complex task at hand. And yet, from the simultaneous repetitive performance of simple behaviours by a large number of individuals, complex patterns emerge. This has come to be known as self-organisation.
The simplest example of self-organisation concerns how ants make the seemingly intelligent decision of choosing the shortest out of many available paths to a food source. Scientists have shown that ants can accomplish this feat without any of the ants making any measurements of path lengths, any knowledge of the advantage of using the shortest path or by actively choosing the shortest path. The ants only need to follow two simple rules: lay a pheromone (volatile chemicals) trail and follow the trails of others.
In the beginning, ants can simply come out of their nest and choose any path randomly if none are already marked with pheromone. Because ants choosing the shortest path (just by chance) will return sooner and thus make more round-trips per unit time, the shortest path will receive the highest amount of pheromone and become more attractive to subsequent ants. In this way, the shortest path will get chosen by all ants in the course of time.
The ants’ brains need to only be as complex as to permit them to lay pheromones and follow the pheromone trails laid by others. The ant colony behaves intelligently without any of the ants being intelligent enough to do so.
There are several obvious advantages of such swarm intelligence. It is flexible because the colony can quickly adapt to changing circumstances. It is robust because the strategy will work even if a few ants fail. And it requires no top-down control and, of course, no great intelligence. In other words, it can be relatively easily genetically programmed by using simple, robotic and repetitive behaviour routines, much like instructions fed into a computer. Not surprisingly, swarm intelligence has become the favoured explanation for the complex behaviours and accomplishments of insect societies and other small-brained animals. It is not difficult to see why it has also found favour with the automation and robotics community.
Pierre-Paul Grassé and stigmergy
One of the first to propose the idea of simple robotic behaviour routines as the explanation for complex behaviour in social insects was the French zoologist and entomologist Pierre-Paul Grassé (1895-1985). Grassé, an expert on termites and a famous supporter of the Lamarckian idea of evolution, was the author of over 300 publications, including the influential 38-volume Traité de Zoologie.
In 1959, Grassé introduced the concept of stigmergy, which has become the organising principle for understanding the so-called “coordination paradox” – i.e., how simple, apparently uncoordinated activities of individuals result in apparently coordinated actions of the group, leading to complex patterns.
The term stigmergy is derived from the Greek roots stigma and ergon. Here stigma refers variously ‘to sting, mark, puncture or tattoo’ – anything that leaves a mark where one individual has already worked. And ergon means ‘work’ or ‘action’. Thus, stigmergy refers to the process by which an individual resumes the work of a previous individual, carrying on from the place where the predecessor left a mark.
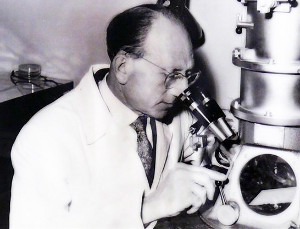
A frequently cited analogy is the laying of bricks for the construction of a wall. Most bricklayers don’t need to know much more than how to lay more bricks in the same pattern as was done by their predecessors. One can see why this is so convenient for programming robots. Of course, there would be problems at the beginning and end of the wall, or if the wall has to be curved etc., but we will return to that later.
Stigmergy has reigned supreme in our exploration of the genesis of complex behaviours from the actions of simple agents, in the context of social insects and beyond, and it has achieved great success. Stigmergy is the leading explanatory framework for studying nest-building behaviour in wasps, bees and termites, and also in studying the behaviour of fish schools and bird flocks.
Guy Theralauz, research director at the University of Toulouse, France, and an academic grandson of Pierre-Paul Grassé, is now at the forefront of applying stigmergic principles to understand the collective behaviour of animals.
But stigmergy has breached the animal behaviour barrier and is now being touted as a universal coordination mechanism with applications in a wide variety of cases, “from chemical reactions to bodily coordination and Internet-supported collaboration in Wikipedia.”
In the case of the honey bee combs, stigmergy and the related concepts of self-organisation have been used to explain not only the hexagonal patterns of the individual cells but also in the large-scale pattern of concentric arrangement of brood cells in the centre, pollen cells in the middle and honey cells in the periphery.
Is stigmergy adequate?
Recall that we lauded stigmergy and the resulting self-organisation as an elegant solution to the problem of how simple brains can make complex patterns. Self-organisation has been such a runaway success that we should turn around and ask if it is really an adequate explanation. And to do so requires the mind of a rebel.
Scientists who go against the prevailing fashion and look for every possible opportunity to overturn well-established theories are often frowned upon as a nuisance unnecessarily rocking the boat. But it is precisely these troublemakers who can potentially bring about what Thomas Kuhn called a paradigm shift in science. And if they fail to bring about a paradigm shift, there is no great harm. Indeed, the prevailing paradigm becomes even stronger every time it survives an assault.
Scientists always prefer the simplest possible theory, with the least number of assumptions, to explain all the facts. This is sometimes referred to as the principle of parsimony and sometimes as Ockham’s razor, attributed to William of Ockham (1287-1347), an English Franciscan friar, scholastic philosopher, and theologian. However, Ockham’s razor can sometimes become tyrannical, and we may fail to discover that real life is indeed more complex than the simplest theory might suggest.
The problem is especially acute where there is often a relentless pressure to not attribute any kind of intelligence, motives, planning, foresight or consciousness to animals. As a result, we may mistakenly treat animals as more robotic and mechanical than they really are.
When I was a graduate student and young researcher, I took great pleasure in cheering Donald R. Griffin (1915-2003), who led a revolt against the prevailing oppressive restrictions on attributing any form of awareness or consciousness to animals. Griffin was well-placed to lead the revolt. He was rightly perceived as a star ethologist for having discovered echolocation in bats and for writing a widely admired book, Listening in the Dark (1958). In a series of books – The Question of Animal Awareness (1976), Animal Thinking (1984) and Animal Minds (1992) – Griffin relentlessly argued in favour of giving animals a chance to demonstrate their true cognitive abilities, the nature of their intelligence and the limits of their consciousness.
In a tribute in 2005, I wrote:
“By and large Griffin’s writings have met with harsh criticism on the grounds that he has failed to produce any definite evidence, ‘the smoking gun’, for his beliefs. Undaunted, Griffin carried on till the very end of his life, and herein lies an important lesson for the scientific community. If some of us are inspired to persist in studying what we believe is important in the face of widespread criticism, Donald Griffin’s efforts would not have gone in vain.”
I am now happy to see that we have at least one worthy torchbearer continuing Griffin’s legacy. Lars Chittka, a professor of sensory and behavioural ecology at the Queen Mary University, London, is today’s leading exponent of the mind of the bee. His book with that title is due to be published in spring 2022. Chittka has conducted outstanding original research on sensory ecology and cognitive behaviour in honey bees and bumblebees. Chittka’s passion for music and the arts combines well with his message that bees may be smarter than we think.
Chittka has collaborated with the musicians Katie Green and Rob Alexander to form the band ‘Killer Bee Queens’, and with the installation artist Julian Walker to raise awareness about the potential of bees to teach humans about our capacity for art and aesthetics.
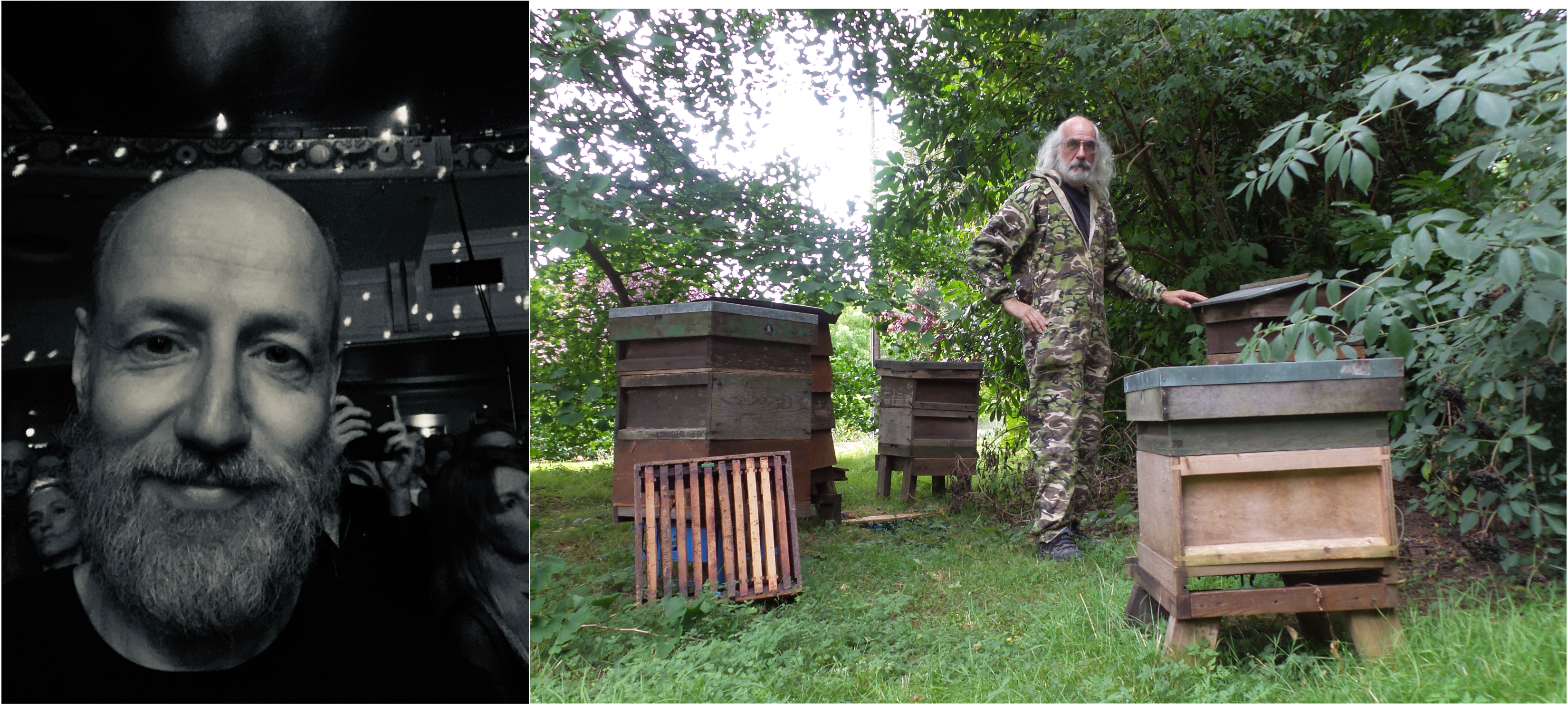
Looking back 200 years
Recently, Chittka teamed up with his PhD student Vincent Gallo to invent yet another strategy to lobby for honey bee cognition. Now, Gallo is by no means a run-of-the-mill PhD student. He recently told me in an email:
“I am a retired software developer and used to specialise in information security, working mainly for clients in banking and government. Once retired, I spent much of my time writing projects that interested me, one of which, based on my amateur beekeeping, was to build a bee simulation. As part of my research to understand how my automata should function, I discovered that the use and formation of honeycomb were insufficiently well understood. At that time, Lars [Chittka] was kind enough to let me become a regular visitor, sitting in on his lab meetings. Shortly thereafter, I asked if he would be my supervisor so that I could explore the unanswered aspect of comb construction, and now, some 4 years hence, I am in my final year, writing my dissertation (and hence, I should point out your error in addressing me as Dr. Gallo – hopefully, but not yet :-)”
Gallo and Chittka have now unearthed, translated, and interpreted a two-century-old and largely forgotten work by another clever honey bees enthusiast, François Huber (1750-1831).
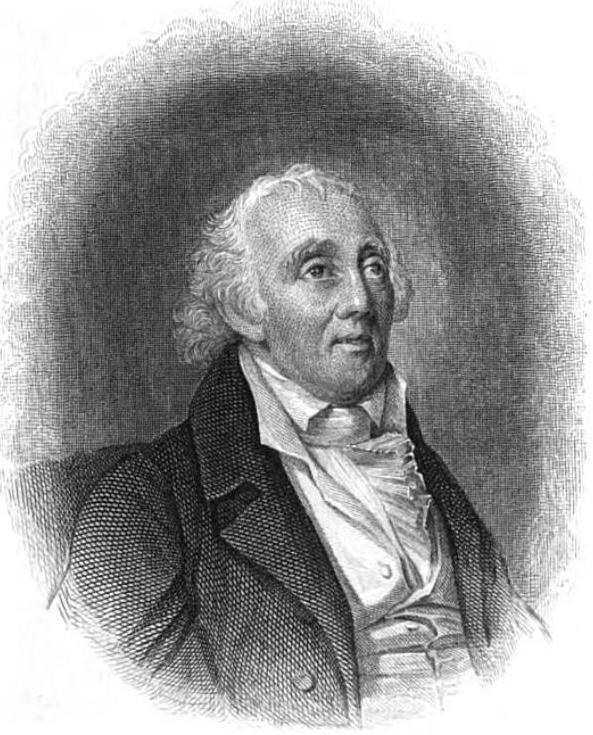
François Huber was quite a well-known scientist of his time who was especially interested in honey bees. He conducted remarkably prescient experiments despite his near blindness for most of his adult life. In a recent paper, entitled ;Cognitive Aspects of Comb-Building in the Honeybee’, published in Frontiers in Psychology, Gallo and Chittka focus on experiments in which Huber reared honey bees in modified hives with glass panes at the top and bottom.
The bees’ wax comb does not stick well to the glass surface, and bees avoided attaching their combs to the glass ceiling. Instead, they built in a very unusual manner starting from the wooden bottom and building upwards. Not satisfied, Huber replaced the wooden base of the hive also with glass. Now, the bees attached their comb to the wooden wall on one side and continued building laterally until they reached the other wooden wall on the other side.
Still not satisfied, Huber placed a glass barrier between the laterally growing comb and the intended endpoint. Even before they hit the glass barrier, the bees curved their comb to attach it to another portion of the same wooden side.
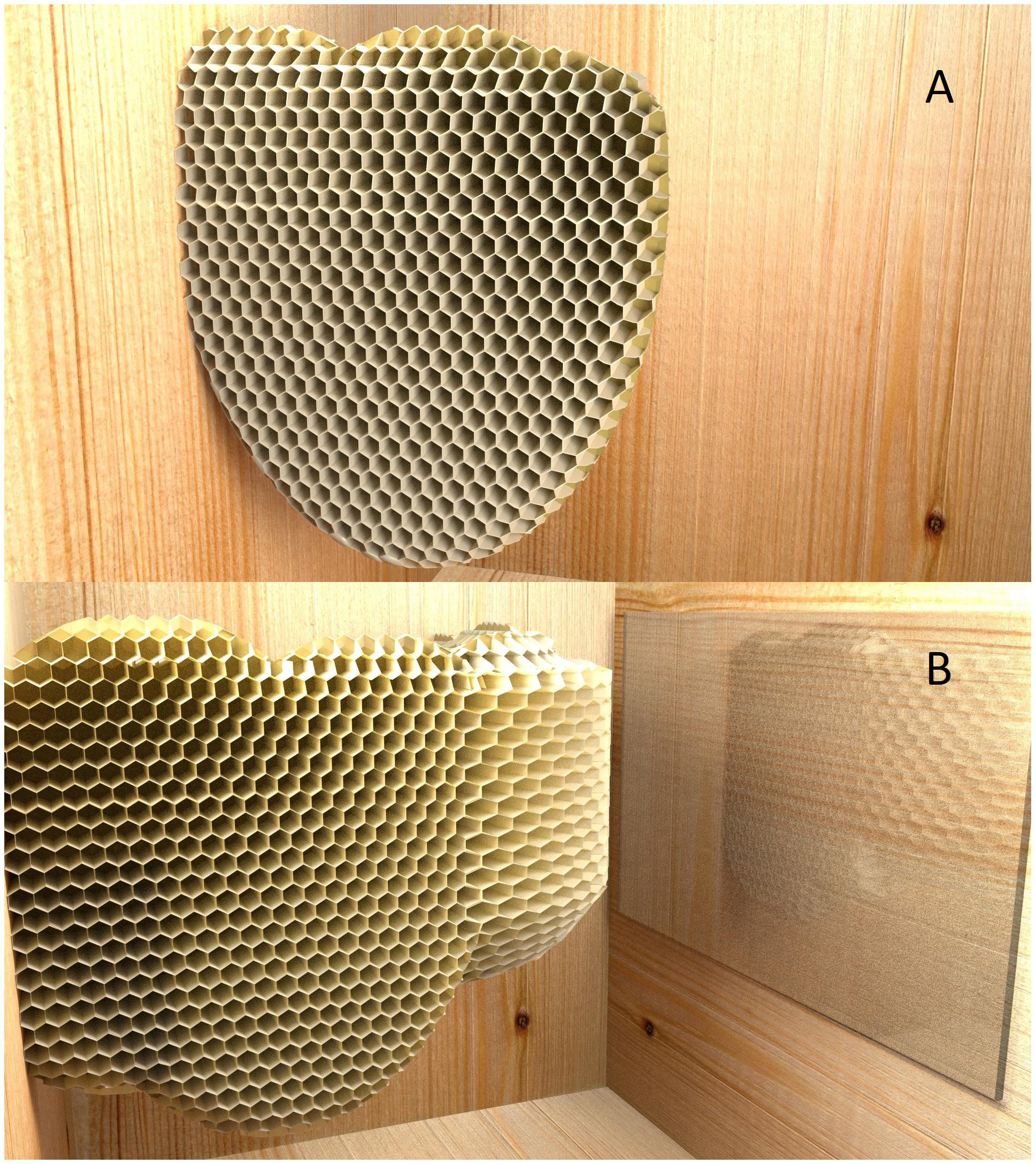
The remarkable response of the bees suggests a great deal of flexibility in their building behaviour. Far from being mere robotic automatons, the bees even seem to be able to plan ahead as they curved their comb even before reaching the glass barrier. Gallo and Chittka concluded:
“Huber’s classic work suggests that honeybees, rather than building wax comb in the way a robot might, may possess a “master plan” of the desired outcome and can tailor their efforts to achieve this goal. Such an interpretation is consistent with recent explorations of intentionality or consciousness-like phenomena in bees.”
Imperfect comb construction
More recently, Michael L. Smith and his colleagues at Auburn University, Alabama, and the Max Planck Institute for Animal Behaviour in the University of Konstanz, Germany, have embarked on yet another novel approach to uncover the extent of the bees’ ability to be flexible and innovate during nest construction.
They capitalise on naturally occurring circumstances where simple stigmergy may not be adequate. One of these circumstances arises when the combs, initiated by different groups of bees, have to be merged to make a single comb. Another is when the bees have to transition from making relatively small cells suitable for rearing worker-destined larvae to slightly large cells suitable for rearing drone-destined larvae.
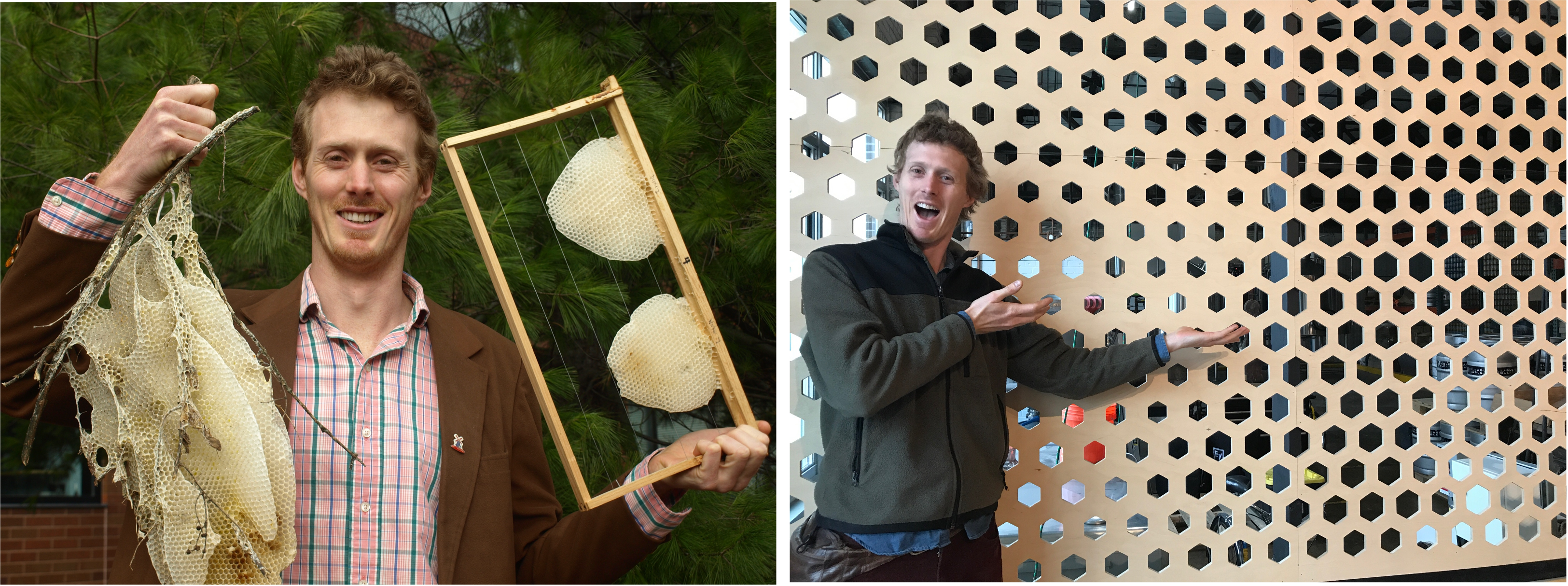
Smith’s method of doing his science is worth noting. He told me in an email:
“My PhD work was focused on how colonies detect the point at which they can “afford” to switch from worker comb to drone comb, so I spent a lot of time looking at comb. Looking at all those combs, I frequently noticed all the non-hexagonal shapes that the bees would build, but I also noticed many examples of the combs merged flawlessly together. That was what really inspired my work, but I knew I needed some new tools to be able to extract useful information. I actually tried measuring cells by hand, with callipers, and I was able to measure quite a few combs, but in doing so, I quickly realized I would need a more efficient way of doing it. For a single cell, there are so many things you might want to measure, and I was hoping to really extract lots of measurements.“
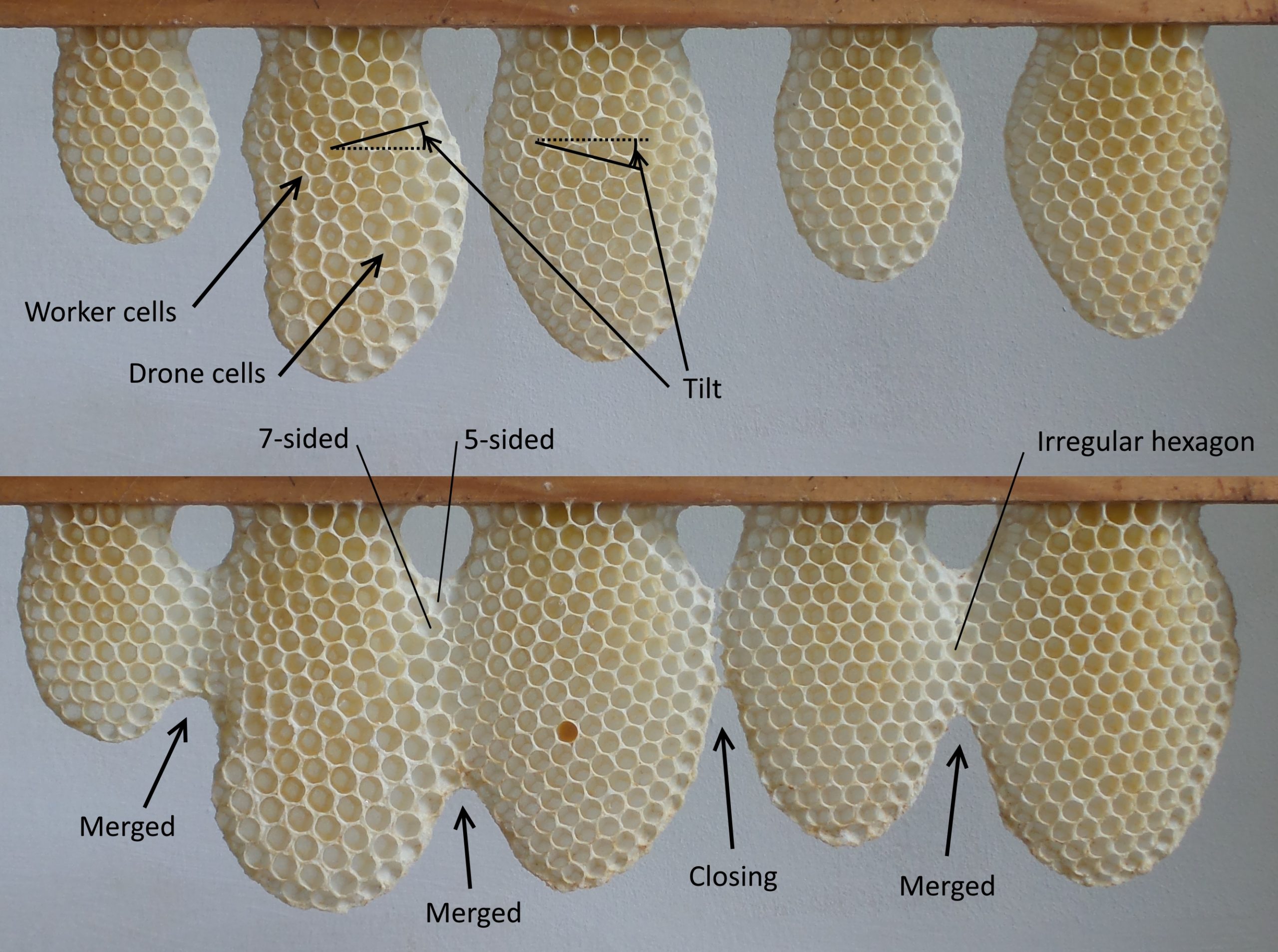
By collaborating with Nils Napp and Kirstin H. Petersen of the Department of Electrical and Computer Engineering, Cornell University, Smith measured multiple parameters of 12,000 cells from natural honey bee combs. They used a combination of automation and human supervision to obtain the large samples while guarding against machine errors.
They found that while honey bees normally make regular hexagonal cells, they make some irregular cells as well – pentagons, heptagons, and also four-, eight- and nine-sided cells, when faced with the unusual task of merging two combs or transitioning from one cell size to another. However, the bees deviated from the preferred hexagons to the least extent required to accomplish their task. But some planning must be involved as they build some intermediate-sized cells before achieving the new cell size required.
Smith and his colleagues also constructed two kinds of computer models – one that was static, to capture the limitations of stigmergic inflexible behaviour, and another a bit more dynamic, to incorporate some planning and some knowledge of the intended goal. Comparing the detailed measurements of the cells made by the real bees and by the two kinds of models, they found that the honey bees outperformed the static model and underperformed the dynamic model.
As Gallo and Chittka comment, these studies by Smith and his colleagues “provide useful input to the question of how much complexity can be achieved by simple rules and the extent to which these might have to be paired with some form of blueprint of the desired outcome to generate functional constructions”.
Thus, bees appear to use stigmergy laced with a bit of cognitive behaviour – mostly Pierre-Paul Grassé but laced with a little bit of François Huber! That’s our conclusion, at least for now. All science is, of course, a work in progress. Who knows what new discoveries the Smiths of the future will make or what past research the Gallos and Chittkas of the future will unearth!
Note: This article was updated at 5:25 pm on September 29, 2021, to correctly attribute two statements to Lars Chittka. They had previously been wrongly attributed to Gallo and Smith.
Raghavendra Gadagkar is a Department of Science and Technology (DST) Year of Science Chair Professor at the Centre for Ecological Sciences at the Indian Institute of Science, Bengaluru.