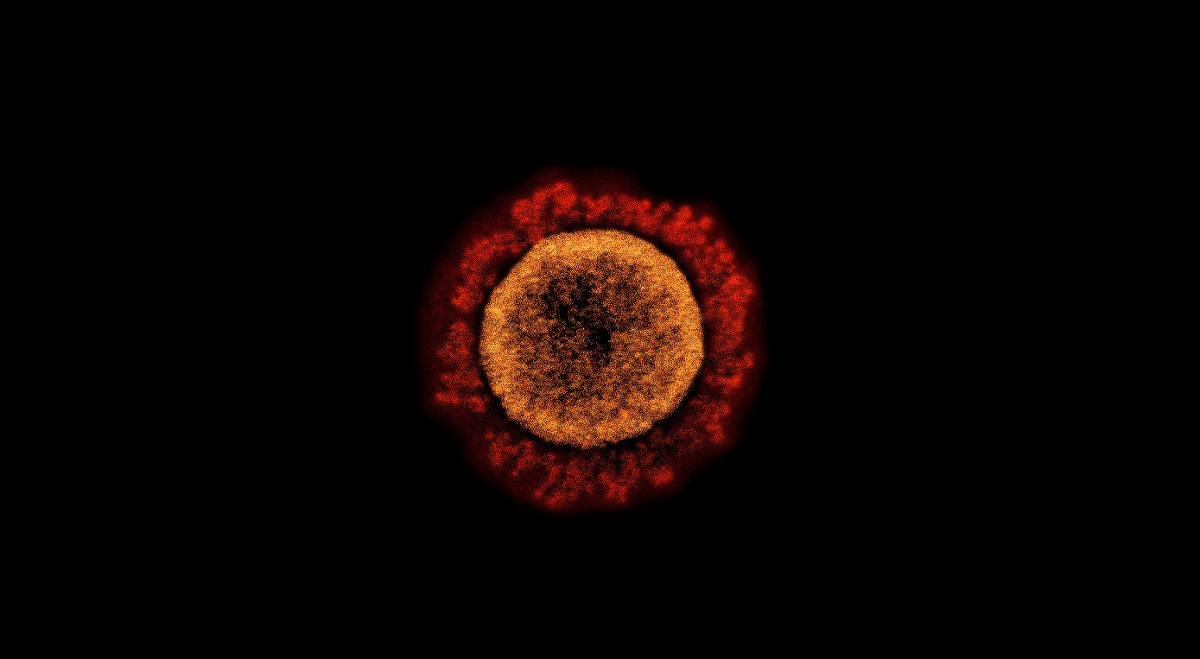
Transmission electron micrograph of a SARS-CoV-2 virus particle. Photo: NIAID/Flick CC BY 2.0
- The common perception is that viruses are sometimes living and at other times non-living, that they are very small, and that they cause disease in humans and animals.
- None of these definitions is wrong – but they are also not entirely correct, and have evolved with time and increasing knowledge.
- Exploring this further is both fun and intellectually satisfying – more so when someone paints a bigger picture to emphasise how viruses are a part of nature’s balance.
- Pranay Lal has done just that in his new book, Invisible Empire – The Natural History of Viruses, writes virologist Shahid Jameel.
When teaching about viruses, I often ask students to define a virus. The most common perceptions are that viruses are sometimes living and at other times non-living, that they are very small, and that they cause disease in humans and animals. None of these definitions is wrong but they are also not entirely correct, and have evolved with time and increasing knowledge.
Exploring this further is both fun and intellectually satisfying – more so when someone paints a bigger picture to emphasise how viruses are a part of nature’s balance, spanning the past, the present and the future. Pranay Lal has done just that in his wonderful new book, Invisible Empire – The Natural History of Viruses.
What fundamentally defines life? Is it the ability to move, consume energy, sense the surroundings, all of these, or something else? Life makes more life that is like itself. Reproduction, therefore, fundamentally defines a living organism. Viruses can make more viruses when they infect another living organism, but not otherwise. They are obligate parasites. Just like every living organism, viruses also carry a genome, which can be either DNA or RNA, and which encodes proteins as functional units.
Being very small, viruses face several structural and functional constraints. A small particle can only accommodate a small genome and the conservation of sequence space demands that the particle is produced with one or just a few proteins. This produces symmetry and the beautiful structures associated with viruses.
The simplest virus needs just one protein (capsid) to make the shell that protects the genome as it goes from one host to another, sometimes making itself evident by causing disease. This possibly prompted biologists Jean and Peter Medawar to remark that a virus is “simply a piece of bad news wrapped up in protein”.
The simplest known viruses, however, make three types of proteins – the capsid to protect the genome, the replicase to make copies of the genome [footnote]DNA viruses don’t need this as they can use polymerases from host cells[/footnote], and protein(s) that can modulate the host. The last one is rarely recognised but is as important from an evolutionary perspective.
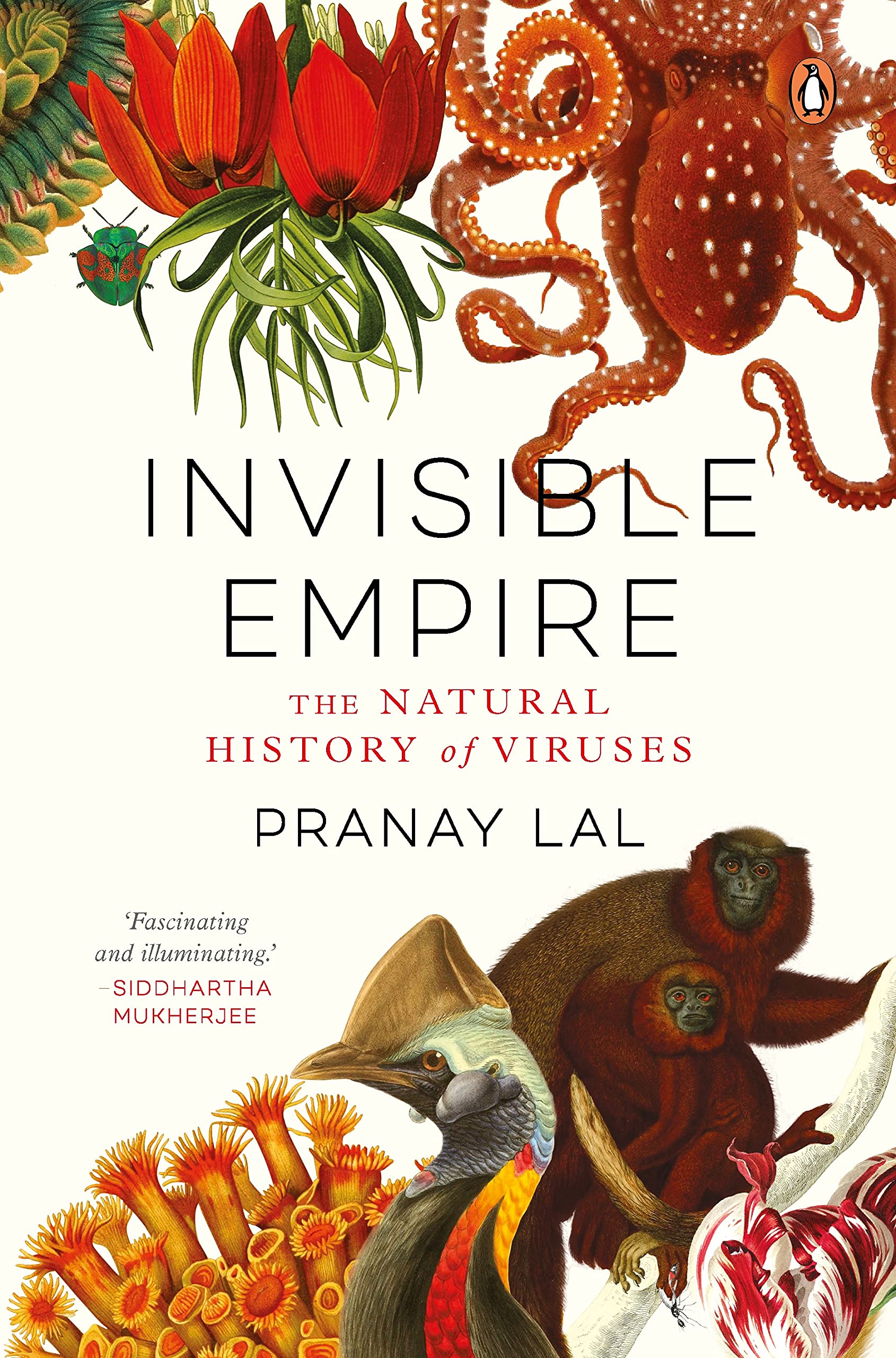
Pranay Lal
Penguin Random House India, October 2021
Just as the population, high rates of replication and random mutations produce a diversity that favours viruses, the hosts and their cells have also evolved strategies to limit virus infection and replication. Unless that is overcome, at least in the short-term, viruses cannot infect productively, multiply, diversify and evolve.
The most common questions about viruses are about what they do, their origins and how they evolve. Reproduction is a fundamental property of life, and all viruses exist to make more viruses. That can occasionally be harmful to the host by killing or transforming the cells in which they multiply, and manifest as disease. Though disease can sometimes help the virus find new hosts and spread better, for example when we cough and sneeze to spread around respiratory viruses, severe disease and a dead host are also a dead end for the virus.
After all, the dead can’t cough, sneeze or have sex. Severe disease often indicates an evolving virus-host relationship that is yet to reach equilibrium.
The origins of viruses are hard to explain because there are no archival records (viruses leave no fossils) and the evolutionary history that can be recovered from phylogenetic analysis has largely eroded, particularly for RNA viruses that evolve much faster than their DNA carrying cousins. Can we use protein structures to predict common ancestry? De novo protein structure predictions using artificial intelligence tools, such as Deep Mind’s AlphaFold, may allow this in future.
Two main hypotheses attempt to describe the genesis of RNA and DNA viruses. The first suggests that viruses have a precellular origin from the primordial RNA world, and the second proposes viruses to have evolved as “escaped genes” from cellular organisms that acquired a capsid and the ability to replicate autonomously. A third suggestion, following the discovery of large DNA viruses, is that cellular organisms shed genes that became obligate parasites.
Whatever be the case, these are not mutually exclusive and viruses could have arisen via more than one pathway. These are described and connected very well in chapter 3 (Supersize Me) of Lal’s book.
Like all life forms, viruses also evolve through mutations and selection. Mutation rates vary quite a bit between viruses, and reflect the enzymes used for genome replication. Viral RNA-dependent RNA polymerases and reverse transcriptases have no proof-reading function – but cellular (and viral) DNA-dependent DNA polymerases do. As a result, mutation rates range between 10-6 to 10-4 substitutions per nucleotide per cell infection (s/n/c) for RNA viruses, 10-5 to 10-3 s/n/c for retroviruses and 6×10-8 to 10-6 s/n/c for DNA viruses.
Selection pressures, such as a different host and immunity, allow some random mutations to carry forward, while the others disappear. There is also an inverse relationship between the mutation rate and the genome size, but the limited range suggests competing evolutionary forces to be at play.
Very low mutation rates provide insufficient beneficial mutations and very high mutation rates lead to excessive mutational load – one is a matter of fidelity, the other fitness. Importantly, due to high rates of replication, viral evolution can be observed on our time scale. This should be evident from numerous SARS-CoV-2 variants we have observed over the last two years.
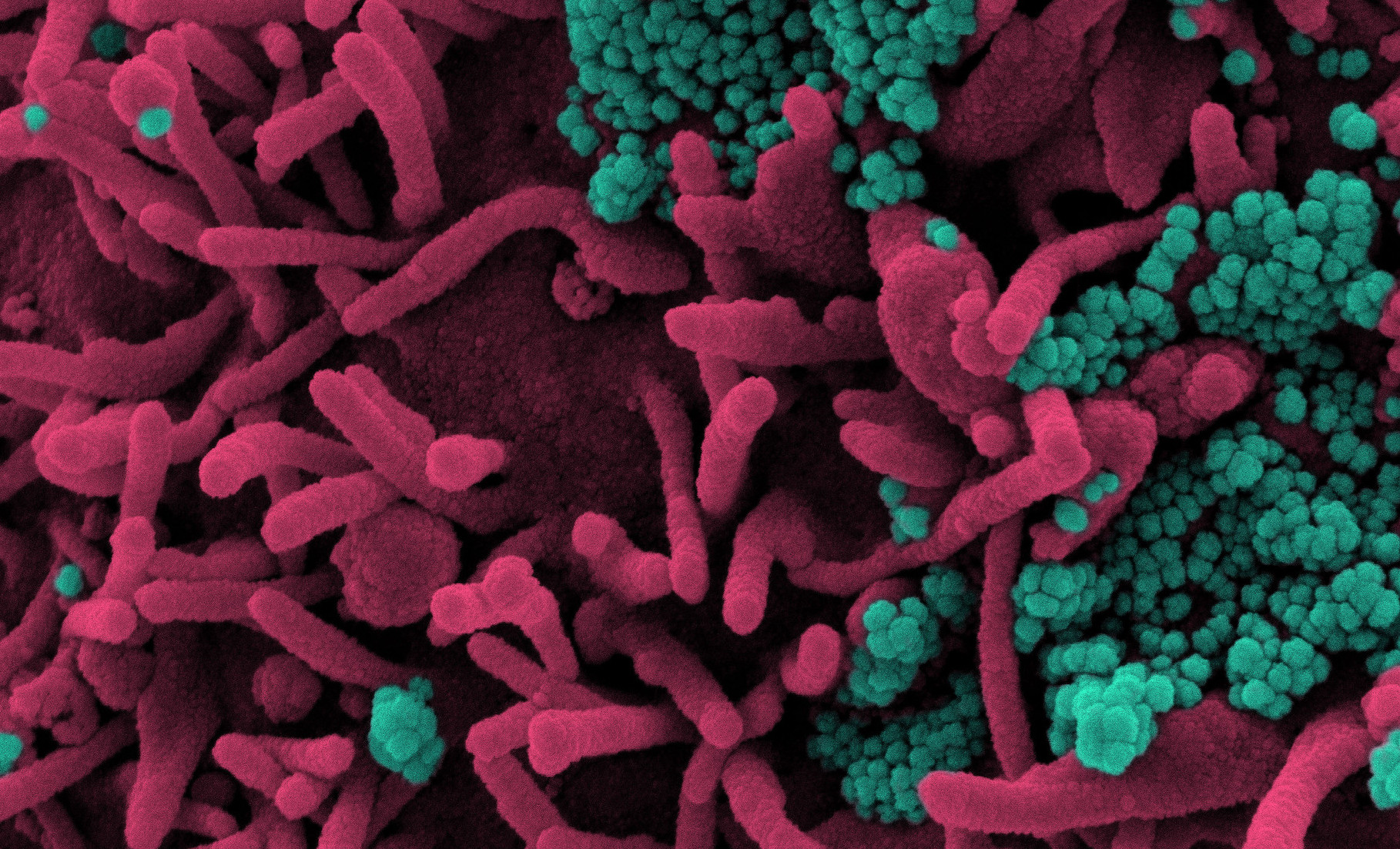
Virologists increasingly specialise in their favourite virus and in time lose perspective of the beauty and diversity that viruses offer, and the broader lessons of evolution and life.
Though I learned much from reading Invisible Empire, it was Lal’s broad-brush view, which paints viruses as essential and fascinating parts of the biosphere, that I enjoyed the most. It was hard to decide which chapter I liked the most, but I will settle for chapter 10 (Beauty), which connects potyviruses, a family of plant viruses, to tulip flower patterns, a medieval craze and trade; and chapter 12 (Zombies), which talks about brain- (or is it mind-?) altering pathogens.
The wasp and cockroach story in this chapter, and how a virus allows the wasp eggs to overcome the cockroach’s immunity, is fascinating. How a fungus can affect an entire ecosystem and how a virus can keep it in check is equally interesting (chapter 11: How A Virus Saved A Giant).
Invisible Empire is as much for the specialist as for the lay reader. While the latter can enjoy the easy prose and the intermittent humour, such as the comparison of British and Indian bureaucracy, p. 156), the specialist can also go to the voluminous Notes (pp. 201-248) for original references. The book is richly illustrated, with expansive figure legends that are themselves a treat to read. Except the occasional ones, like those on pages 67, 82-83 and 96-97, where the combination of font size and colour, make it hard on the eyes.
In all earnestness, I must also point out two minor bloopers to be corrected in future editions. On page 10, hepatitis E virus is called a Togavirus, but it is now classified as a Hepevirus. And on page 99, the DNA base pairing is mentioned to be through a phosphate link, when it should have been hydrogen bonding.
One can’t write about viruses today without commenting on the ongoing pandemic. What lessons have we learned for the future? It is said that COVID-19 didn’t break the system, but simply exposed how broken the system really was. It also exposed what we call “normal” to be very abnormal.
A looming climate crisis will not just change weather patterns and bring droughts and floods; it will also bring new diseases. The thawing permafrost and release of anthrax spores from an infected animal causing an outbreak in Siberia in 2016, the 2020 discovery of 28 novel groups of viruses from 15,000-year-old glacial ice cores, and modelling studies on the survival of Aedes mosquitos that show the risk of first exposure to a mosquito borne disease for over one billion people by 2080, are all cause for worry. Public health must become a priority, and needs to move from a ‘reaction’ to a ‘preparation’ mode.
Joshua Lederberg, who won the Nobel Prize for his work on microbial genetics at age 33 and then lived to the ripe age of 84 years, was possibly the most prominent biologist and thinker of our times. In a 1989 speech, he argued how life is a dense web of mutualism and genetic interactions that provides abundant sources of genetic variation “for viruses to learn new tricks” and become “man’s only real competitors”.
Pranay Lal’s Invisible Empire – The Natural History of Viruses follows in the same vein. It is now recommended reading for my students.
Shahid Jameel is a virologist, who is also interested in travel, history and photography. He is presently a fellow at OCIS and Green Templeton College, University of Oxford, UK; and a visiting professor at Ashoka University, India. He set up the Virology Group at the International Centre for Genetic Engineering and Biotechnology, New Delhi, India, in 1988, and led it for the next 25 years.