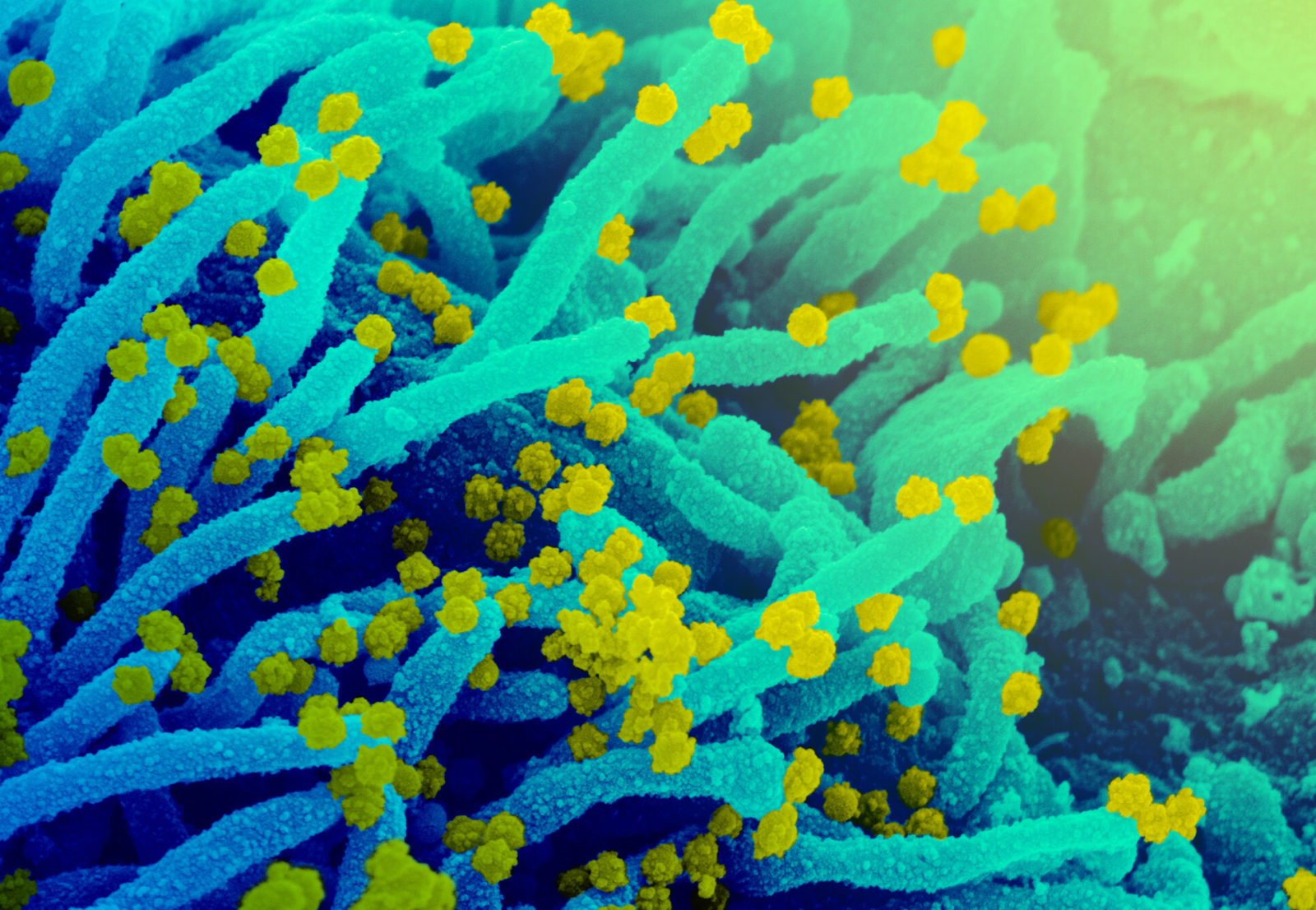
A scanning electron microscope image of SARS-CoV-2 (round yellow particles) emerging from the surface of a cell cultured in the lab. Image: NIAID
Variants of SARS-CoV-2, the coronavirus that causes COVID-19, have emerged and threaten to interminably prolong the pandemic. The major variant, delta, that seems to have caused the second wave in India recently, is still going strong, with experts fearing that we may be on the cusp of a third wave.
An increasing number of SARS-CoV-2 genome sequences are becoming available at a rapid pace and new potentially worrying variants are being identified. What are these variants and why do they emerge? What is their impact on the pandemic? How effective are the currently authorized vaccines against them? Scientists are addressing these questions while rapidly accumulating new information on the variants, their genetic sequences and their properties.
A genome and its mutations
SARS-CoV-2 stores its genetic information in a chemical substance called RNA. This RNA is a long polymeric molecule assembled from four kinds of building blocks, known as nucleotides, represented by the letters A, G, C and U. It is approximately 30,000 letters long. The precise order of these letters represents the virus’s genetic information, and is organised into segments called genes, along the length of the RNA molecule. The RNA molecule with all the genes in it is known as the virus’s genome.
Each gene encodes information for the virus to create a gene product with a specific function. Collectively, these functions are orchestrated to build more SARS-CoV-2 particles inside the infected cells of our body. Proteins are one class of gene products. They are polymers built of 20 different chemical building blocks known as amino acids. For example, the S gene encodes the gene product known as the spike protein, which the virus uses to enter susceptible cells in the human body.
A change in the genetic information is known as a mutation. This may be the removal or addition of one or more letters, or replacement of one or more letters by other letters. When SARS-CoV-2 multiplies in an infected cell, its RNA is used to make hundreds of thousands of new RNA copies, which get packaged into progeny viruses. During such copying, errors can creep in, making mutations a natural byproduct of viral multiplication. Certain mutations may help the virus in some way, giving it an edge and allowing it to out-compete other viruses lacking such mutations.
Early on during the pandemic, SARS-CoV-2 showed limited diversity – that is, not many mutations were evident. With increasing spread of SARS-CoV-2 to more and more people as the pandemic progressed, there was a concomitant increase in the opportunity for virus multiplication in new hosts and the accumulation of worrisome mutations.
In other words, the more the virus circulates, the more it evolves. The scope for virus multiplication and the associated opportunity for the emergence of mutations are likely to be heightened in individuals whose immune systems may be weak due to age, illness or because of specific therapies.
Also read: New Covaxin Study Makes Absence of Phase 3 Data More Conspicuous
How are these mutations identified?
Scientists can identify the mutations acquired by SARS-CoV-2 genes over time by looking at the sequence of the letters in the individual genes. This process is called genome-sequencing. Sequencing a large number of virus samples collected from an exposed population in different geographic locations, at different times, reveals information about how the genetic information of the virus changed over time. This is known as genomic surveillance. It helps scientists monitor the changes in the virus’s genome as it evolves during the pandemic.
A particular mutation may confer an element of fitness to the virus. For example, it may enhance the capacity of the virus to multiply, spread or slip past our immune system. Obviously, viruses carrying such advantageous mutations will tend to persist in the population. On the other hand, a mutation that may make the virus less fit will be lost eventually. Biologists call this process natural selection, which largely dictates the evolution of the virus. This is the same concept that Charles Darwin put forth in his seminal work On The Origin of Species (1859).
Scientists have discovered many such fitness-conferring mutations in SARS-CoV-2. While mutations may occur in many genes, S gene mutations are of interest because the spike protein it encodes is critical in the ability of SARS-CoV-2 to enter cells. And all vaccines aim to teach our immune systems to recognise it as a danger signal to fight the virus.
Spike mutations
The S gene of SARS-CoV-2 encodes the genetic information to make the spike protein, a long chain of 1,273 amino acids. This chain folds into a unique shape; three spike protein molecules assemble together in a trimeric arrangement, to create one spike protuberance on the surface of the virus. This spike trimer is the part of the virus that recognises and attaches to the ACE2 receptor (an enzyme) on the surface of susceptible human cells.
Many single-letter changes of significant concern have been identified on the spike protein. One of the earliest mutations to be recognised was a single change in the S gene whose effect was to replace the 614th amino acid, known as aspartic acid (denoted by D) with another amino acid called glycine (G). This change is called the D614G mutation. It changes the shape of the spike protein in a subtle way, making it more stable. Scientists have found that viruses with the D614G mutation spread faster because they are more efficient at infecting cells. In other words, it is more transmissible. But it does not seem to be associated with greater disease severity or escaping immunity.
Other notable spike mutations include the replacement of the amino acids asparagine (N) by tyrosine (Y) at the 501st position (N501Y), and lysine (K) by either asparagine or threonine (T), at the 417th position (K417N, K417T). Because of the way the spike protein folds, both amino acids at positions 417 and 501 are located at the tip of the spike. These mutations are thought to allow a better and tighter fit with the ACE2 receptor.
Another mutation, which replaces glutamic acid (E) with lysine (K) at position 484 (E484K), changes the shape of the spike protein in a way that likely allows it to escape from certain antibodies. (Antibodies are proteins, produced by an infected or vaccinated person’s immune system, which attach to the virus and help destroy it). The K417N mutation also appears to help the virus evade some antibodies.
Also read: Worrying That COVID-19 Cases Plateauing, Not Declining: Ashish Jha
SARS-CoV-2 variants
The study of disease in populations in terms of its frequency, pattern, causes, risk factors, and control strategies is known as epidemiology. In the past several months, the communication and discussion on SARS-CoV-2 epidemiology has been dominated by variants. A mutation refers to the actual change in nucleotide sequence in the gene, which in turn is reflected by a change in amino acid sequence of the encoded protein. A virus carrying one or more mutations in its genetic material is called a variant.
Most SARS-CoV-2 variants identified so far actually possess a constellation of mutations. The particular set of mutations associated with a given variant collectively contributes to its overall characteristics. Many SARS-CoV-2 variants that are cause for worry, as they may prolong the pandemic, are known as ‘variants of concern’.
During the course of the pandemic, scientists observed four epidemiological events in Britain, South Africa, Brazil and India, during May-October 2020. Each of these events was associated with a particular cluster of variant SARS-CoV-2 sequences. Each variant cluster represented a distinct lineage. Recently the WHO introduced a simple way to name these variants, using the Greek alphabet: alpha, beta, gamma and delta. All of them carry the D614G spike mutation, in addition to other mutations, and are all regarded as variants of concern.
The Alpha variant, first detected in Britain in September 2020, has since been detected in over 150 countries. It has the D614G and N501Y mutations, as well as P681H and a couple of double amino-acid deletions at positions 69-70 and 144-145. A recent report suggested that the alpha variant is not only more transmissible but also capable of causing more severe illness.
The beta variant, first detected in May 2020 in South Africa, has since spread to nearly 100 countries. The notable spike mutations it contains are N501Y, K417N and E484K. The beta variant appears to be able to dodge vaccine-induced immunity.
The gamma variant, first identified in Brazil in November 2020, has until now been reported from 60 countries. It shares many of the same spike mutations with the beta variant, and is consequently suspected of being able to evade vaccine-induced immunity.
The delta variant, first identified in India in October 2020, has been reported from 92 countries. It carries more than a dozen mutations, of which two are significant: E484Q and L452R. The former is thought to help the variant escape certain antibodies and the latter to help it spread more efficiently. The delta variant is regarded as being more contagious than the alpha variant.
A recent report on genomic surveillance in India showed that the delta variant is currently the predominant driver of COVID-19 outbreaks in the country. Delta’s prevalence rose from ~10% of all samples sequenced in February 2021 to ~90% by the end of May 2021, and has been linked to the country’s second wave.
Recently, scientists identified another variant, which has a K417N mutation (seen in the beta variant) in addition to all the other delta mutations. This has been called delta plus, and currently represents ~0.1% of sequenced samples. Its impact, if any, is expected to be seen in the coming months.
The emergence of variants with better ability to infect, spread and even evade immunity can complicate our efforts to contain the pandemic. The more SARS-CoV-2 circulates, the more it can evolve, giving rise to more variants. In fact, several additional variants, considered to be of interest, have already been identified and designated the Greek letters epsilon to lambda. Scientists are following them to see if they eventually become variants of concern.
Can vaccines overcome the variants?
Arguably, the most effective way to end the pandemic would be to prevent the emergence and spread of all possible variants. How well does the current crop of authorised vaccines work against the variants of concern?
Limited information is available on the impact of the alpha, beta and delta variants on the performance of some COVID-19 vaccines. Not much information is available for vaccine performance against the gamma variant either. It is important to emphasise that the available information on variants and how they affect vaccine performance is limited as well as preliminary. In addition, information about one COVID-19 vaccine against a variant cannot be extrapolated to other vaccines and variants.
The Pfizer, Moderna and the Johnson & Johnson vaccines appear to be effective against both the alpha and beta variants in protecting against symptomatic as well as severe disease. The Pfizer and AstraZeneca (known as Covishield in India) vaccines appear to protect against severe but not symptomatic disease caused by the delta variant.
The AstraZeneca vaccine was notably ineffective against symptomatic disease linked to the beta variant.
Protection against symptomatic disease, offered by the Bharat Biotech vaccine Covaxin, was also blunted by the Delta variant.
Also read: Bharat Biotech Releases Long-Awaited Covaxin Phase 3 Data, Claims 77.8% Efficacy
The available information suggests that while the Pfizer vaccine can offer good protection against infection by the alpha variant, its efficacy against preventing infection by the beta and delta variants is much lower. The AstraZeneca vaccine may be effective in preventing infection by the alpha, but not by the beta and delta variants.
Note that in a situation in which several variants are circulating in a population, and when this population begins to be vaccinated, the vaccine-susceptible variants will be gradually eliminated but the vaccine-resistant variants will continue to multiply, leading to a situation in which the prevalent variants in the population are the vaccine-resistant ones. This can favour the emergence and dominance of resistant variants, akin to the emergence of antibiotic resistance.
The good news is that most of the vaccines in use have demonstrated (in clinical trials) the ability to prevent severe COVID-19 and death with great efficacy – and these are also outcomes linked to many variants of concern. So achieving widespread vaccine coverage can minimise the risk of severe COVID-19 and death.
But overall, we need vaccines not just to eliminate the virus but also to completely block transmission of all possible variants. At present, we do not know how efficacious the current COVID-19 vaccines are on this count. And until a current or future vaccine is found to be an ‘all variants’ transmission-blocking vaccine, the hope of returning to pre-pandemic life may continue to be a distant one.
S. Swaminathan is a retired scientist based in Hyderabad. The views expressed here are the author’s own.